Brain Structural Abnormalities in Psychotropic Drug-Naive Pediatric Patients With Obsessive-Compulsive Disorder
Abstract
OBJECTIVE: The authors investigated structural abnormalities in brain regions comprising cortical-striatal-thalamic-cortical loops in pediatric patients with obsessive-compulsive disorder (OCD). METHOD: Volumes of the caudate nucleus, putamen, and globus pallidus and gray and white matter volumes of the anterior cingulate gyrus and superior frontal gyrus were computed from contiguous 1.5-mm magnetic resonance images from 23 psychotropic drug-naive pediatric patients with OCD (seven male patients and 16 female patients) and 27 healthy volunteers (12 male subjects and 15 female subjects). RESULTS: Patients had smaller globus pallidus volumes than healthy volunteers, but the two groups did not differ in volumes of the caudate nucleus, putamen, or frontal white matter regions. Compared to healthy volunteers, patients had more total gray matter in the anterior cingulate gyrus but not the superior frontal gyrus. Total anterior cingulate gyrus volume correlated significantly and positively with globus pallidus volume in the healthy volunteers but not in patients. CONCLUSIONS: These findings provide evidence of smaller globus pallidus volume in patients with OCD without the potentially confounding effects of prior psychotropic drug exposure. Volumetric abnormalities in the anterior cingulate gyrus appear specific to the gray matter in OCD, at least at the gross anatomic level, and are consistent with findings of functional neuroimaging studies that have reported anterior cingulate hypermetabolism in the disorder.
Obsessive-compulsive disorder (OCD) has a lifetime prevalence rate of 2%–3% in the general population (1) and is marked by significant and often chronically disabling functional impairment. OCD is considered the ninth leading cause of disability in the United States and other countries (2). Because OCD may have pediatric onset in as many as 80% of patients (3), investigation of its neurobiology may be facilitated by studying the illness in childhood, when the potentially confounding variables of illness duration and prior pharmacologic exposure are less likely to be present than they would be in adulthood.
Abnormal feedback loops within cortical-striatal-thalamic-cortical circuits have been hypothesized to play a key role in the pathophysiology of OCD. Specifically, a defect in the basal ganglia’s ability to provide sensory gating by filtering and suppressing inputs from cortical regions has been considered important to OCD phenomenology (4–8). Basal ganglia abnormalities have been linked to OCD from studies that have demonstrated obsessive-compulsive symptoms in individuals with known dysfunction in these regions (4). Several prior structural magnetic resonance imaging (MRI) studies of the caudate nucleus have reported no volume differences between patients and healthy volunteers (9–13), although others have reported larger (14) or smaller (15) volumes in patients. Studies of other parts of the corpus striatum, including the putamen (13, 16) and globus pallidus (16–18), in OCD have also yielded mixed findings.
Neurobiological models of OCD have also emphasized abnormalities in the frontal lobes (6–8). In particular, the anterior cingulate basal ganglia-thalamocortical circuit (19–21) has been hypothesized to play a key role in compulsive behavior (4). Evidence from neuropsychological (22), structural MRI (23), and functional neuroimaging (24, 25) studies has implicated anterior cingulate abnormalities in the pathogenesis of OCD. Moreover, a developmentally mediated network dysplasia involving the basal ganglia and anterior cingulate has been hypothesized to disrupt brain functions that mediate ongoing purposive behavior in OCD (13). In contrast to the attention focused on the anterior cingulate gray matter, there has been little systematic study of the anterior cingulate white matter in OCD, despite its strong connections with other brain regions that have also been implicated in the pathophysiology of OCD, including the ventromedial caudate, putamen, and nucleus accumbens.
We performed a volumetric MRI study to test the hypothesis that pediatric patients with OCD would differ from healthy volunteers in volumes of cortical and subcortical regions that comprise cortical-striatal-thalamic-cortical circuits. Given that there is little evidence for superior frontal gyrus abnormalities in OCD (16, 23, 26), we used this brain structure as a comparison region to determine specificity of anatomic pathology. To rule out potential effects of illness chronicity and medication on the observed findings, we studied only psychotropic drug-naive patients early in the course of illness.
Method
Subjects
Twenty-three psychotropic drug-naive pediatric outpatients with OCD and 27 healthy comparison subjects participated in this study (Table 1). This group of subjects did not overlap with the group of subjects in our previous research in this area (13, 23). Eight patients had comorbid anxiety disorders, three had dysthymia, one had oppositional defiant disorder, one had attention deficit disorder without hyperactivity, two had trichotillomania, and 11 had OCD as their only diagnosis. Their mean age at illness onset was 9.1 years (SD=2.7).
All patients were recruited through the child psychiatry outpatient clinic at Wayne State University School of Medicine in Detroit. The patients’ diagnoses were made by using DSM-IV criteria and the Schedule for Affective Disorders and Schizophrenia for School-Age Children—Present and Lifetime Version (27). All subjects and their parents were interviewed by a child and adolescent psychiatrist with board certification (D.R.R.). Exclusion criteria for patients and healthy comparison subjects included lifetime history of unipolar or bipolar disorder, psychosis, eating disorders, substance abuse or dependence, Sydenham’s chorea, Tourette’s disorder, other tic-related conditions, conduct disorder, significantly debilitating medical or neurological conditions, pervasive developmental disorder, mental retardation, or learning disorder. There was no history of psychiatric illness in the healthy comparison subjects or in any of their first-degree relatives. The child’s parents served as informants. Legal guardians provided written informed consent, and all subjects provided written assent.
Clinical Assessments
OCD symptom severity was assessed in all subjects with the Children’s Yale-Brown Obsessive Compulsive Scale (28, 29). The 17-item Hamilton Depression Rating Scale (30) was used to measure severity of depression, and the Hamilton Anxiety Rating Scale (31) was used to measure severity of anxiety. Tic severity was measured with the Yale Global Tic Severity Scale (32).
MRI Procedures
MRI examinations were conducted at the Children’s Hospital of Michigan Imaging Center by using image acquisition methods described previously (33). Briefly, MRI images were acquired in the coronal plane by using a three-dimensional spoiled gradient echo pulse sequence with a 40° flip angle, 25-msec repetition time, and 5-msec echo time on a 1.5-T whole-body superconducting imaging system (General Electric, Milwaukee). This sequence produced 124 contiguous coronal slices (slice thickness=1.5 mm) through the whole head with nominal in-plane resolution of 0.94×0.94 mm in a 256×256 matrix. Axial proton density and T2-weighted images were obtained to exclude visually detectable structural abnormalities on MRI scans.
Measurements were completed in the MEDx program (34) after alignment along the anterior and posterior commissures for purposes of standardization. Before all measurements, the MRI images of the patients and the comparison subjects were flipped randomly in the right-left axis and mixed together. No identifying information was available from the images. All measurements were completed in the coronal plane by well-trained and reliable operators (S.M., S.C., K.B., D.H.) who were blind to the subject’s group membership and hemisphere.
Intracranial Volume
National Institutes of Health image software (version 1.61) (35) was used to compute intracranial volume in a manner described previously (13). This technique yields valid and reliable neuroanatomical measurements by using a semiautomated segmentation approach (36).
Regions of Interest
Frontal lobe subregions
Detailed methods for measuring the anterior cingulate gyrus and superior frontal gyrus have been described previously (37). The boundaries of the anterior cingulate gyrus were (anterior, posterior, ventral, dorsal): the tip of the cingulate sulcus, the connection of the superior and precentral sulci, the callosal sulcus, and the cingulate sulcus. The boundaries of the superior frontal gyrus were (anterior, posterior, lateral, medial): the tip of the cingulate sulcus, the connection of the superior and precentral sulci, the superior frontal sulcus, and the cingulate sulcus. Frontal regions of interest were segmented by using a thresholding algorithm that was generated from gray-level histograms (38) and has been used in our previous work (39).
Caudate nucleus
Measurement began when the caudate was first visible rostrally, and care was taken to exclude cerebrospinal fluid medially and to exclude the nucleus accumbens ventrally. Lateral projections of the caudate were included in the measurements. The last slice measured was the point at which the tail of the caudate was no longer clearly visible. We distinguished between the caudate and the accumbens primarily by comparing the voxel intensities of these structures and their shape along the rostrocaudal axis. The nucleus accumbens was first observed on the ventral part of the caudate around the first appearance of the putamen and formed a bridge of tissue between the caudate and lenticular nucleus.
Putamen
Measurement began when the putamen was first visible rostrally as an oval structure. Caudally, the putamen was separated from the caudate nucleus by the internal capsule, the nucleus accumbens, and then the globus pallidus, which became the medial boundary. The ventral border was the anterior commissure and then later the anterior perforated substance. Dorsally, the putamen was separated from the caudate by part of the anterior and then the posterior limb of the internal capsule. Measurement was discontinued when the putamen was no longer visible in the coronal plane.
Globus pallidus
Measurement began at the first appearance of the globus pallidus rostrally. The dorsomedial border was the internal capsule, and the lateral border was the putamen. On the most rostral slices, the anterior commissure was used as the ventral border and the medial border was the point at which the internal capsule and anterior commissure appeared to intersect. More caudally, the substantia innominata was the ventral border and the ansa lenticularis was included in the measurement. On the most caudal slices, the substantia innominata remained the ventral border. Medially, the border was the internal capsule and/or the crus cerebri. Both the internal and external globus pallidus were included in the volume.
Reliability
Intraclass correlations between two or three operators for measurement of eight to 10 cases were (right hemisphere, left hemisphere): 0.91 and 0.95 for the caudate nucleus, 0.91 and 0.86 for the putamen, 0.84 and 0.87 for the globus pallidus, 0.90 and 0.94 for the anterior cingulate gyrus gray matter, 0.94 and 0.94 for the anterior cingulate gyrus white matter, 0.92 and 0.97 for the superior frontal gyrus gray matter, and 0.95 and 0.95 for the superior frontal gyrus white matter. The intraclass correlation for intracranial contents was 0.99.
Data Analysis
Group differences in demographic variables were examined by using t tests for independent samples. Chi-square tests were used to examine differences in joint classifications of discrete variables. Associations between continuous variables were examined by using Pearson’s product-moment correlations.
The main statistical analysis involved multivariate analysis of covariance (MANCOVA), which tested for an overall difference in brain structure volumes between the psychotropic drug-naive patients and the healthy comparison subjects. Age and intracranial volume served as statistical covariates. We also considered the possible effects of anxiety and depression on brain structure volumes by including these variables as statistical covariates. All analyses were two-tailed; alpha was set at 0.05.
Results
The characteristics and clinical assessment data for the psychotropic drug-naive patients with OCD and the healthy comparison subjects are summarized in Table 1. All patients had significant dysfunction as measured by the Children’s Yale-Brown Obsessive Compulsive Scale score (mean=28.6, range=17–40). The patients and the comparison subjects did not differ significantly in distributions of age, sex, parental socioeconomic status, or handedness (all p>0.05). The groups did not differ significantly in intracranial volume (patients: mean=1188 cm3, SD=109; healthy volunteers: mean=1169 cm3, SD=112) (t=0.60, df=48, p=0.55).
Because the group-by-hemisphere interaction was not significant for any of the brain structure volumes, right and left hemisphere volumes were pooled for the analyses. In addition, given that the diagnosis-by-sex interaction was not significant for any of the brain structure volumes (p>0.05 for all analyses), sex was not included in the final model. The MANCOVA for the brain structure volumes revealed that the overall main effect of diagnosis was significant (Wilks’s lambda=0.69, F=2.56, df=7, 40, p<0.03), indicating that the volumes differed between the groups. Univariate tests revealed that the patients had more anterior cingulate gyrus gray matter (F=5.68, df=1, 46, p<0.03) and smaller volumes of the globus pallidus (F=5.06, df=1, 46, p<0.03), compared to the healthy volunteers. Significant group differences were still evident when the effects of depression and anxiety were controlled. In addition, patients who had a comorbid diagnosis (N=12) did not differ significantly from patients who had OCD as their sole diagnosis (N=11) in either anterior cingulate gyrus gray matter or globus pallidus volumes (p>0.05). The mean brain structure volumes are presented in Table 2, as are the adjusted 95% confidence intervals for the differences between group means (the mean for the healthy comparison group minus the mean for the patient group). The distributions of the unadjusted anterior cingulate gyrus gray matter and globus pallidus volumes by group are illustrated in Figure 1 and Figure 2, respectively.
We found no significant correlations of Children’s Yale-Brown Obsessive Compulsive Scale, Hamilton depression scale, or Hamilton anxiety scale scores with either anterior cingulate gray matter volume or globus pallidus volume in the patients (p>0.05). However, globus pallidus and total anterior cingulate gyrus volumes were positively correlated in the healthy comparison subjects (r=0.51, df=27, p=0.007) but not in the patients (r=–0.07, df=23, p=0.75).
Discussion
Our findings provide additional evidence for brain structural abnormalities in the pathophysiology of OCD without the potentially confounding effects of prior psychotropic drug exposure and chronicity of illness. To our knowledge, these findings provide the first controlled MRI evidence of structural alterations in the globus pallidus in OCD. In addition, using methods for cortical parcellation based on the sulcal anatomy, we found that volumetric abnormalities in the anterior cingulate gyrus are specific to the gray matter, at least at the gross anatomic level, in patients, compared to healthy volunteers.
Few MRI studies have investigated the globus pallidus in OCD. Although Jenike et al. (16) did not identify any globus pallidus abnormalities in a group of 10 female adults with OCD, the small number of subjects may have limited the statistical power. Sampling differences, including differences in prior pharmacologic exposure, could also account for discrepant findings. Our findings are consistent with case reports linking globus pallidus pathology to obsessive-compulsive phenomenology (17, 18). Similarly, Laplane et al. (40) reported obsessive-compulsive behavior in seven patients with basal ganglia lesions, five of whom specifically had globus pallidus pathology. Moreover, abnormal metabolic rate in the globus pallidus has also been implicated in the pathophysiology of OCD (41). Prior reports of higher than normal globus pallidus volume in OCD have been linked to streptococcal infection (42) and higher antibody titers (43); these findings are consistent with the hypothesis of an inflammatory autoimmune response. Thus, these earlier findings may not be directly comparable to our findings. Further investigation of the relationship between brain structure volumes and indices of streptococcal infection is warranted.
The globus pallidus has been considered to play an important role in neurobiological models of OCD (5, 6). In particular, this region has considerable afferent connections from other regions implicated in the pathophysiology of OCD, including the thalamus and striatum (44). Moreover, the orbital frontal lobe has extensive connections with the ventromedial caudate and nucleus accumbens, which also project to the ventromedial globus pallidus. In the model by Baxter et al. (6), the orbital frontal lobe is hypothesized to produce “worry” outputs that drive OCD-relevant circuits in the caudate nucleus, thereby increasing inhibitory output to other brain regions, including the globus pallidus. Similarly, Modell et al. (5) have proposed that dysfunction within a fronto-striato-pallido-thalamo-frontal circuit could contribute to the inhibitory mechanisms (i.e., control or loss of control) observed in OCD.
Few structural MRI studies have investigated anatomically relevant frontal lobe subregions in OCD, which may be an important consideration, given that the frontal lobes are both structurally and functionally heterogeneous. Several prior studies in OCD did not report group differences in volumetric measures of the entire prefrontal cortex (13, 15). Jenike et al. (16) reported larger operculum volumes in 10 female patients, compared to 10 healthy volunteers. For the same group of subjects, Grachev et al. (45) reported that the volumes of 10 frontal cortical parcellation units (including the right inferior frontal pars triangularis and the right midfrontal cortex) were larger in patients, compared to healthy volunteers. One advantage of the methods described herein is that they are based on the sulcal anatomy and, thus, may provide greater cytoarchitectonic validity, compared to methods that use invariant landmarks that are not on the cortical surface, such as the corpus callosum. It should be acknowledged, however, that the size of cytoarchitectonic fields can vary among individuals and that these variations may not map neatly onto the sulcal anatomy of the cortex (46). Nevertheless, it is noteworthy that no group differences were observed in a frontal “comparison” region—the superior frontal gyrus—thus demonstrating some degree of specificity of gray matter anatomic pathology to the anterior cingulate gyrus in OCD.
The finding of more anterior cingulate gyrus gray matter in patients is consistent with reports of functional abnormalities in this region (24, 25, 47, 48). Recent functional magnetic resonance imaging data suggest that hyperactivity in the anterior cingulate may be associated with abnormal conflict detection as part of an overactive action monitoring system in OCD (49). Moreover, animal studies suggest that the anterior cingulate plays a key role in reward expectancy (50). Thus, this region may have particular relevance to behavioral theories regarding OCD pathogenesis. More anterior cingulate gray matter in OCD could reflect a neurodevelopmental abnormality that possibly involves neuronal pruning (23), although it is also conceivable that hyperfunctioning in the anterior cingulate could lead to increased gray matter volume.
Our finding of a larger amount of anterior cingulate gray matter in patients replicates prior findings (23) in a new group of psychotropic drug-naive patients and by means of different methods. Our findings extend this prior work by demonstrating that structural alterations in the anterior cingulate gyrus are specific to the gray matter, at least at the gross anatomic level, given the nonsignificant group differences in anterior cingulate white matter volume. If anterior cingulate abnormalities are specific to the gray matter in OCD, this finding may partly explain why we did not observe group differences in volumes of the total (gray plus white) anterior cingulate gyrus in a previous study (37), although the majority of patients in that study had been exposed to psychotropic medications, thus making comparisons with the present findings difficult. In a study by Kim et al. (26), however, that included adults with OCD who had all remained psychotropic drug-free for at least 4 weeks before the MRI scan, no gray matter abnormalities in the anterior cingulate gyrus were identified in patients by means of statistical parametric mapping, suggesting that methodologic and/or sampling issues (i.e., pediatric versus adult patients) may be important considerations in interpreting the study findings. However, the patients in the study by Kim et al. (26) may have required more time for medication washout before specific structural abnormalities in the anterior cingulate could be detected.
Taken together, the findings of abnormalities in both the globus pallidus and the anterior cingulate gyrus in the patients are consistent with the model of OCD pathophysiology described by Rapoport and Wise (4). In this model, striatal cell groups act as signal detectors either for sensory-derived inputs from the cortex or for “internal motivation” inputs from the cingulate cortex. Sensory input to the striatum causes an innately programmed cell group to recognize the input and stop the tonic discharge of pallidal cells. Removal of thalamic inhibition releases thalamocortical circuits, thus leading to a normal behavioral response. If signals from the cingulate cortex to the striatum converge on the same pallidal cell group responsible for the species-typical behavior, then cingulate hyperactivity could conceivably activate this circuit without concomitant sensory input or motivation to perform the behavior, thus causing it to be completed compulsively. The finding of a positive correlation between globus pallidus and anterior cingulate gyrus volumes in the healthy volunteers, but not in the patients, in the present study is consistent with the hypothesis that a defect in connectivity of frontal-subcortical circuitry may play a role in the pathophysiology of OCD.
Similar to some MRI studies (9–13), but in contrast to others (14, 15), our study did not find structural abnormalities in the caudate nucleus of patients with OCD. Differences among studies—including differences in delineation criteria, the number of treated patients among the study subjects, the duration of and type of psychotropic drug exposure, and diagnostic comorbidity—could conceivably lead to discrepant findings. The lack of significant group differences in caudate nucleus volume in our study is consistent with a meta-analysis of the functional neuroimaging literature that found similar caudate functioning in patients with OCD and in healthy comparison subjects after the results were normalized for global metabolism (10). It is also possible that the use of other neuroimaging modalities may be required to detect caudate nucleus pathology in OCD. For example, in a spectroscopy study, Bartha et al. (12) found decreased N-acetylaspartate in the left corpus striatum of patients with OCD without associated group differences in volume, thus highlighting the purported sensitivity of this technique, compared to volumetry.
We did not replicate prior findings of smaller putamen volume in pediatric patients with OCD (13), although the lack of group differences is consistent with a prior study of adult patients (16). We considered whether operator drift could be one possible explanation; however, the average difference between putamen volumes measured twice for 16 subjects was less than 1%. It is possible that sampling differences between the present study and our prior study may at least partly account for the different findings. For example, 30% of the patients in the current study were male, compared to 68% of the patients in our prior study. The raw data from our prior study (13) show that smaller putamen volume was most evident among male patients, relative to male comparison subjects, an effect that may have been undetected in the present study, given the fewer number of male patients. The patients in the present study were also more severely ill than the patients in our prior study, as reflected by the higher Children’s Yale-Brown Obsessive Compulsive Scale (mean=29 and mean=23, respectively) and Hamilton depression scale (mean=10 and mean=6, respectively) scores of the patients in the present study. These differences suggest that the patient groups in the two studies may not be directly comparable. Finally, methodologic differences in defining the putamen also may have contributed to the differences in findings.
In summary, we have reported the results of a structural MRI study in a new cohort of psychotropic drug-naive pediatric patients with OCD. The findings provide additional evidence for involvement of frontal and subcortical brain regions in the pathophysiology of OCD without the potentially confounding effects of exposure to psychotropic medication. Future studies with the goal of cross-validating the present findings by means of different research techniques, such as voxel-based morphometry, would be useful.
![]() |
![]() |
Presented in part at the annual meeting of the American College of Neuropsychopharmacology, San Juan, Puerto Rico., Dec. 8–12, 2002. Received July 29, 2003; revision received Oct. 7, 2003; accepted Oct. 9, 2003. From the Department of Psychiatry Research, Zucker Hillside Hospital, North Shore-Long Island Jewish Health System; the Department of Psychiatry, Albert Einstein College of Medicine, Bronx, N.Y.; the Departments of Psychiatry and Behavioral Neurosciences, Radiology, and Pediatrics, Wayne State University School of Medicine, Detroit; and the Department of Psychiatry, University of Minnesota, Minneapolis. Address reprints to Dr. Szeszko, Department of Psychiatry Research, Zucker Hillside Hospital, 75-59 263rd St., Glen Oaks, NY 11004; [email protected] (e-mail). Supported in part by grants from the National Alliance for Research on Schizophrenia and Depression (Dr. Szeszko, Dr. Rosenberg), NIMH grant MH-01990 (Dr. Szeszko), NIMH grants MH-59299, MH-65122, and MH-02037 (Dr. Rosenberg), the Miriam L. Hamburger Endowed Chair at the Children’s Hospital of Michigan and Wayne State University (Dr. Rosenberg), and the State of Michigan Joe F. Young Sr. Psychiatric Research and Training Program. The authors thank Denise Head for assistance in image analysis.
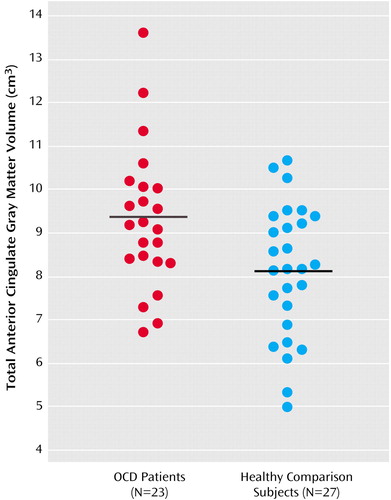
Figure 1. Total Unadjusted Anterior Cingulate Gyrus Gray Matter Volume in Psychotropic Drug-Naive Pediatric Patients With Obsessive-Compulsive Disorder (OCD) and Healthy Comparison Subjectsa
aHorizontal lines represent mean values.
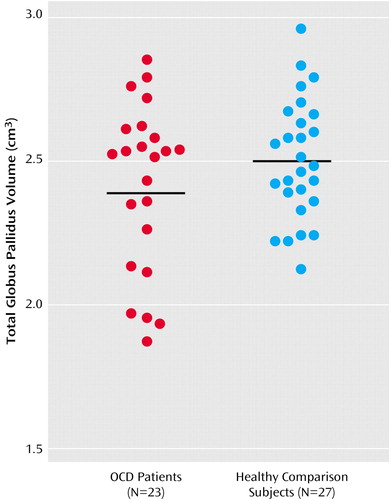
Figure 2. Total Unadjusted Globus Pallidus Volume in Psychotropic Drug-Naive Pediatric Patients With Obsessive-Compulsive Disorder (OCD) and Healthy Comparison Subjectsa
aHorizontal lines represent mean values.
1. Rasmussen SA, Eisen JL: The epidemiology and differential diagnosis of obsessive compulsive disorder. J Clin Psychiatry 1992; 53(April suppl):4–10Google Scholar
2. Murray CJL, Lopez AD: The Global Burden of Disease. Boston, Harvard University Press, 1996Google Scholar
3. Pauls DL, Alsobrook JP II, Goodman W, Rasmussen S, Leckman JF: A family study of obsessive-compulsive disorder. Am J Psychiatry 1995; 152:76–84Link, Google Scholar
4. Rapoport JL, Wise SP: Obsessive-compulsive disorder: evidence for basal ganglia dysfunction. Psychopharmacol Bull 1988; 24:380–384Medline, Google Scholar
5. Modell JG, Mountz JM, Curtis GC, Greden JF: Neurophysiologic dysfunction in basal ganglia/limbic striatal and thalamocortical circuits as a pathogenetic mechanism of obsessive-compulsive disorder. J Neuropsychiatry Clin Neurosci 1989; 1:27–36Crossref, Medline, Google Scholar
6. Baxter LR, Schwartz JM, Bergman KS, Szuba MP, Guze BH, Mazziotta JC, Alazraki A, Selin CE, Ferng HK, Munford P, Phelps MD: Caudate glucose metabolite changes with both drug and behavior therapy for obsessive-compulsive disorder. Arch Gen Psychiatry 1992; 49:681–689Crossref, Medline, Google Scholar
7. Baxter LR Jr: Positron emission tomography studies of cerebral glucose metabolism in obsessive compulsive disorder. J Clin Psychiatry 1994; 55(Oct suppl):54–59Google Scholar
8. Insel TR: Toward a neuroanatomy of obsessive-compulsive disorder. Arch Gen Psychiatry 1992; 49:739–744Crossref, Medline, Google Scholar
9. Aylward EH, Schwartz J, Machlin S, Pearlson G: Bicaudate ratio as a measure of caudate volume on MR images. AJNR Am J Neuroradiol 1991; 12:1217–1222Medline, Google Scholar
10. Aylward EH, Harris GJ, Hoehn-Saric R, Barta PE, Machlin SR, Pearlson GD: Normal caudate nucleus in obsessive-compulsive disorder assessed by quantitative neuroimaging. Arch Gen Psychiatry 1996; 53:577–584Crossref, Medline, Google Scholar
11. Kellner CH, Jolley RR, Holgate RC, Austin L, Lydiard RB, Laraia M, Ballenger JC: Brain MRI in obsessive-compulsive disorder. Psychiatry Res 1991; 36:45–49Crossref, Medline, Google Scholar
12. Bartha R, Stein MB, Williamson PC, Drost DJ, Neufeld RWJ, Carr TJ, Canaran G, Densmore M, Anderson G, Siddiqui AR: A short echo 1H spectroscopy and volumetric MRI study of the corpus striatum in patients with obsessive-compulsive disorder and comparison subjects. Am J Psychiatry 1998; 155:1584–1591Link, Google Scholar
13. Rosenberg DR, Keshavan MS, O’Hearn KM, Dick EL, Bagwell WW, Seymour AB, Montrose DM, Pierri JN, Birmaher B: Frontostriatal measurement in treatment-naive children with obsessive-compulsive disorder. Arch Gen Psychiatry 1997; 54:824–830Crossref, Medline, Google Scholar
14. Scarone S, Colombo C, Livian S, Abbruzzese M, Ronchi P, Locatelli M, Scotti G, Smeraldi E: Increased right caudate nucleus size in obsessive-compulsive disorder: detection with magnetic resonance imaging. Psychiatry Res 1992; 45:115–121Crossref, Medline, Google Scholar
15. Robinson D, Wu H, Munne RA, Ashtari M, Alvir JM, Lerner G, Koreen A, Cole K, Bogerts B: Reduced caudate nucleus volume in obsessive-compulsive disorder. Arch Gen Psychiatry 1995; 52:393–398Crossref, Medline, Google Scholar
16. Jenike MA, Breiter HC, Baer L, Kennedy DN, Savage CR, Olivares MJ, O’Sullivan RL, Shera DM, Rauch SL, Keuthen N, Rosen BR, Caviness VS, Filipek PA: Cerebral structural abnormalities in obsessive-compulsive disorder: a quantitative morphometric magnetic resonance imaging study. Arch Gen Psychiatry 1996; 53:625–632Crossref, Medline, Google Scholar
17. Rodrigo Escalona P, Adair JC, Roberts BB, Graeber DA: Obsessive-compulsive disorder following bilateral globus pallidus infarction. Biol Psychiatry 1997; 42:410–412Crossref, Medline, Google Scholar
18. Demirkol A, Erdem H, Inan L, Yigit A, Guney M: Bilateral globus pallidus lesions in a patient with Tourette syndrome and related disorders. Biol Psychiatry 1999; 46:863–867Crossref, Medline, Google Scholar
19. Alexander GE, DeLong MR, Strick PL: Parallel organization of functionally segregated circuits linking basal ganglia and cortex. Annu Rev Neurosci 1986; 9:357–381Crossref, Medline, Google Scholar
20. Alexander GE, Crutcher MD: Functional architecture of basal ganglia circuits: neural substrates of parallel processing. Trends Neurosci 1990; 13:266–271Crossref, Medline, Google Scholar
21. Cummings JL: Frontal-subcortical circuits and human behavior. Arch Neurol 1993; 50:873–880Crossref, Medline, Google Scholar
22. Rauch SL, Whalen PJ, Dougherty D, Jenike MA: Neurobiological models of obsessive-compulsive disorder, in Obsessive-Compulsive Disorders: Practical Management. Edited by Jenike MA, Baer L, Minichiello WE. St Louis, Mosby, 1998, pp 222–253Google Scholar
23. Rosenberg DR, Keshavan MS: AE Bennett Research Award: toward a neurodevelopmental model of obsessive-compulsive disorder. Biol Psychiatry 1998; 43:623–640Crossref, Medline, Google Scholar
24. Swedo SE, Schapiro MB, Grady CL, Cheslow DL, Leonard HL, Kumar A, Friedland R, Rapoport SI, Rapoport JL: Cerebral glucose metabolism in childhood-onset obsessive-compulsive disorder. Arch Gen Psychiatry 1989; 46:518–523Crossref, Medline, Google Scholar
25. Rauch SL, Jenike MA, Alpert NM, Baer L, Breiter HC, Savage CR, Fischman AJ: Regional cerebral blood flow measured during symptom provocation in obsessive-compulsive disorder using oxygen 15-labeled carbon dioxide and positron emission tomography. Arch Gen Psychiatry 1994; 51:62–70Crossref, Medline, Google Scholar
26. Kim JJ, Lee MC, Kim J, Kim IY, Kim SI, Han MH, Chang KH, Kwon JS: Gray matter abnormalities in obsessive-compulsive disorder: statistical parametric mapping of segmented magnetic resonance images. Br J Psychiatry 2001; 179:330–334Crossref, Medline, Google Scholar
27. Kaufman J, Birmaher B, Brent D, Rao U, Flynn C, Moreci P, Williamson D, Ryan N: Schedule for Affective Disorders and Schizophrenia for School-Age Children—Present and Lifetime Version (K-SADS-PL): initial reliability and validity data. J Am Acad Child Adolesc Psychiatry 1997; 36:980–988Crossref, Medline, Google Scholar
28. Goodman WK, Price LH, Rasmussen SA, Mazure C, Fleischmann RL, Hill CL, Heninger GR, Charney DS: The Yale-Brown Obsessive Compulsive Scale, I: development, use, and reliability. Arch Gen Psychiatry 1989; 46:1006–1011Crossref, Medline, Google Scholar
29. Wolff RP, Wolff LS: Assessment and treatment of obsessive-compulsive disorder in children. Behav Modif 1991; 15:372–393Crossref, Medline, Google Scholar
30. Hamilton M: Development of a rating scale for primary depressive illness. Br J Soc Clin Psychol 1967; 6:278–296Crossref, Medline, Google Scholar
31. Hamilton M: The assessment of anxiety states by rating. Br J Med Psychol 1959; 32:50–55Crossref, Medline, Google Scholar
32. Leckman JF, Riddle MA, Hardin MT, Ort SI, Swartz KL, Stevenson J, Cohen J: The Yale Global Tic Severity Scale: initial testing of a clinician-rated scale of tic severity. J Am Acad Child Adolesc Psychiatry 1989; 28:566–573Crossref, Medline, Google Scholar
33. Gilbert AR, Moore GJ, Keshavan MS, Paulson LA, Narula V, MacMaster FP, Stewart CM, Rosenberg DR: Decrease in thalamic volumes of pediatric patients with obsessive-compulsive disorder who are taking paroxetine. Arch Gen Psychiatry 2000; 57:449–456Crossref, Medline, Google Scholar
34. MEDx. Sterling, Va, Sensor Systems, 1998Google Scholar
35. Rasband W: National Institutes of Health Image Manual. Bethesda, Md, NIH, 1996Google Scholar
36. Keshavan MS, Beckwith C, Bagwell W, Pettegrew JW, Krishnan KRR: An objective method for edge detection in MRI morphometry. Eur Psychiatry 1994; 9:205–207Google Scholar
37. Szeszko PR, Robinson D, Alvir JM, Bilder RM, Lencz T, Ashtari M, Wu H, Bogerts B: Orbital frontal and amygdala volume reductions in obsessive-compulsive disorder. Arch Gen Psychiatry 1999; 56:913–919Crossref, Medline, Google Scholar
38. Otsu N: A threshold selection method from gray-level histograms. IEEE Trans Syst Man Cybern 1979; 9:63–66Google Scholar
39. Lim KO, Zipursky RB, Watts MC, Pfefferbaum A: Decreased gray matter in normal aging: an in vivo magnetic resonance study. J Gerontol 1992; 47:B26-B30Google Scholar
40. Laplane D, Levasseur M, Pillon B, Dubois B, Baulac M, Mazoyer B, Tran Dinh S, Sette G, Danze F, Baron JC: Obsessive-compulsive and other behavioural changes with bilateral basal ganglia lesions: a neuropsychological, magnetic resonance imaging and positron tomography study. Brain 1989; 112(part 3):699–725Google Scholar
41. McGuire PK, Bench CJ, Frith CD, Marks IM, Frackowiak RS, Dolan RJ: Functional anatomy of obsessive-compulsive phenomena. Br J Psychiatry 1994; 164:459–468Crossref, Medline, Google Scholar
42. Giedd JN, Rapoport JL, Garvey MA, Perlmutter S, Swedo SE: MRI assessment of children with obsessive-compulsive disorder or tics associated with streptococcal infection. Am J Psychiatry 2000; 157:281–283Link, Google Scholar
43. Peterson BS, Leckman JF, Tucker D, Scahill L, Staib L, Zhang H, King R, Cohen DJ, Gore JC, Lombroso P: Preliminary findings of streptococcal antibody titers and basal ganglia volumes in tic, obsessive-compulsive, and attention deficit/hyperactivity disorders. Arch Gen Psychiatry 2000; 57:364–372Crossref, Medline, Google Scholar
44. Carpenter MB: Core Text of Neuroanatomy, 4th ed. Baltimore, Williams & Wilkins, 1991Google Scholar
45. Grachev ID, Breiter HC, Rauch SL, Savage CR, Baer L, Shera DM, Kennedy DN, Makris N, Caviness VS, Jenike MA: Structural abnormalities of frontal neocortex in obsessive-compulsive disorder. Arch Gen Psychiatry 1998; 55:181–182Crossref, Medline, Google Scholar
46. Rajkowska G, Goldman-Rakic PS: Cytoarchitectonic definition of prefrontal areas in the normal human cortex, II: variability in locations of areas 9 and 46 and relationship to the Talairach coordinate system. Cereb Cortex 1995; 5:323–337Crossref, Medline, Google Scholar
47. Breiter HC, Rauch SL, Kwong KK, Baker JR, Weisskoff RM, Kennedy DN, Kendrick AD, Davis TL, Jiang A, Cohen MS, Stern CE, Belliveau JW, Baer L, O’Sullivan RL, Savage CR, Jenike MA, Rosen BR: Functional magnetic resonance imaging of symptom provocation in obsessive-compulsive disorder. Arch Gen Psychiatry 1996; 53:595–606Crossref, Medline, Google Scholar
48. Perani D, Colombo C, Bressi S, Bonfanti A, Grassi F, Scarone S, Bellodi L, Smeraldi E, Fazio F: [18F]FDG PET study in obsessive-compulsive disorder: a clinical/metabolic correlation study after treatment. Br J Psychiatry 1995; 166:244–250Crossref, Medline, Google Scholar
49. Ursu S, Stenger VA, Shear MK, Jones MR, Carter CS: Overactive action monitoring in obsessive-compulsive disorder: evidence from functional magnetic resonance imaging. Psychol Sci 2003; 14:347–353Crossref, Medline, Google Scholar
50. Shidara M, Richmond BJ: Anterior cingulate: single neuronal signals related to degree of reward expectancy. Science 2002; 296:1709–1711Crossref, Medline, Google Scholar