Psychiatric Applications of Repetitive Transcranial Magnetic Stimulation
Abstract
Principles of TMS
Stimulation Target
Stimulation Parameters
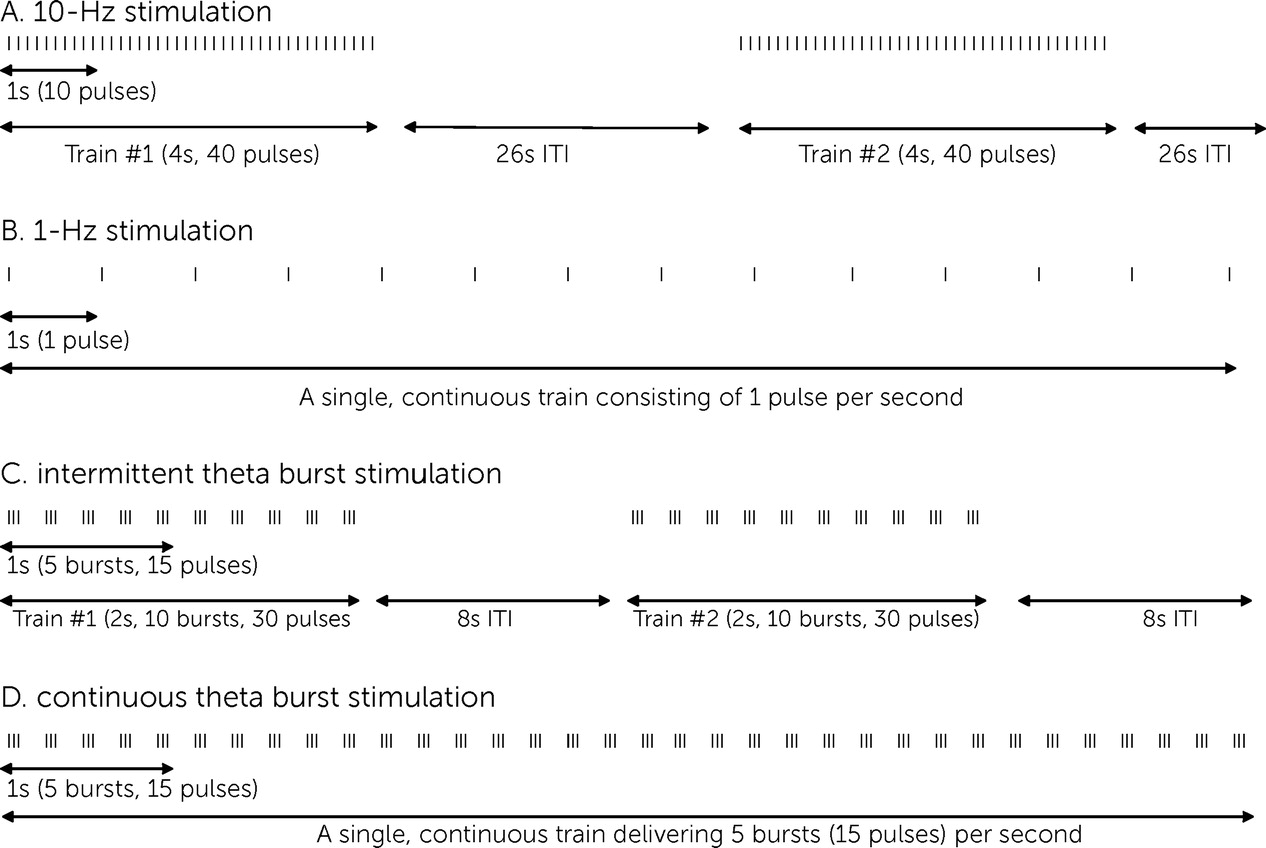
Indications, Protocols, and Outcomes
Overview
Target | Protocol | Typical course | Cleared system(s) (33) | Outcomes |
---|---|---|---|---|
Major depressive disorder | ||||
Left DLPFC | Commonly termed “10 Hz” or “HFL” (high-frequency left). 3,000 pulses are delivered at a frequency of 10 Hz in 75 trains (4-second duration, 40 pulses per train, 26-second ITI). Goal intensity is 120% MT (3). | Sessions last 37.5 minutes. Standard course is 36 sessions: 5 sessions per week for 6 weeks, followed by a 3-week taper (3, then 2, then 1 weekly session). | Apollo TMS, CloudTMS, Magstim, MagVenture, NeuroStar, Nexstim | Response rate of 47%, remission rate of 27% (N=192) in a controlled setting (32). In a naturalistic setting, response rate of 58%, remission rate of 37% (N=307) (34). About 63% of responders (N=120) maintain benefit 12 months later (35). |
Left DLPFC | Identical to HFL, except ITI is reduced to between 11 and 25 seconds (36). | Identical to HFL, except session time is reduced to 19–36 minutes, depending on the selected ITI. | Apollo TMS, CloudTMS, Magstim, MagVenture, NeuroStar, Nexstim | Comparably safe and effective to standard 10 Hz treatment (36). |
Left DLPFC | Commonly termed “theta burst” or “iTBS.” 600 pulses of iTBS delivered as 200 bursts (each consisting of 3 pulses delivered at 50 Hz). Bursts delivered at a frequency of 5 Hz in 2-second trains separated by an 8-second ITI. Goal intensity is 120% MT (32). | Each session is 3 minutes. Standard course of 36 sessions: 5 sessions per week for 6 weeks, followed by a 3-week taper (3, then 2, then 1 weekly session). | BrainsWay with H1-coil, Magstim, MagVenture, NeuroStar, Nexstim | Noninferior to standard 10 Hz treatment; response rate of 49%, remission rate of 32% (N=193) (32). |
Left DLPFC | 1,980 pulses are delivered at a frequency of 18 Hz in 55 trains (2-second duration, 36 pulses per train, 20-second ITI). Goal intensity is 120% MT (37). | Each session is 20 minutes. Typical course involves 4 weeks of acute treatment (5 treatments per week) followed by up to 12 weeks of maintenance treatment (2 treatments per week) (37). | BrainsWay with H1-coil | Response rate of 38%, remission rate of 33% (N=89) with acute treatment (38). Response rates up to 73%, remission rates up to 64% with continued treatment of non-responders (N=33) (39). |
Obsessive-compulsive disorder | ||||
Bilateral DMPFC and ACC | 2,000 pulses delivered at a frequency of 20 Hz in 50 trains (2-second duration, 40 pulses per train, 20-second ITI). Goal intensity is 100% of the leg motor threshold (19, 40). | Each 18-minute session is preceded by 3–5 minutes of symptom provocation. A typical course administers 5 treatments per week for a total of 6 weeks (19, 40). | BrainsWay with H7-coil, MagVenture with Cool D-B80 coil | Response rate of 45%, partial response rate of 60% (N=42) 1 month posttreatment (19). |
Smoking cessation | ||||
Prefrontal cortex and insula | 1,800 pulses delivered at a frequency of 10 Hz in 60 trains (3-second duration, 30 pulses per train, 15-second ITI). Goal intensity is 120% MT (41). | Each 20-minute session is preceded by a brief craving provocation. A typical course of 5 daily sessions for 3 weeks, followed by 1 weekly session for another 3 weeks (41). | BrainsWay with H4-coil | Of highly addicted smokers who completed treatment (N=169), 28% achieved 4 consecutive weeks of abstinence (42). |
Major Depressive Disorder
OCD
Smoking Cessation
Safety Considerations
Electromagnetic Forces
Seizure Risk
Side Effects
Practical Considerations
Evaluation and Monitoring
Concomitant Treatment
Device Selection
Treatment Setting
Personnel and Training
Insurance Coverage
Billing
Future Directions
Conclusions
References
Information & Authors
Information
Published In
History
Keywords
Authors
Competing Interests
Metrics & Citations
Metrics
Citations
Export Citations
If you have the appropriate software installed, you can download article citation data to the citation manager of your choice. Simply select your manager software from the list below and click Download.
For more information or tips please see 'Downloading to a citation manager' in the Help menu.
View Options
View options
PDF/EPUB
View PDF/EPUBLogin options
Already a subscriber? Access your subscription through your login credentials or your institution for full access to this article.
Personal login Institutional Login Open Athens loginNot a subscriber?
PsychiatryOnline subscription options offer access to the DSM-5-TR® library, books, journals, CME, and patient resources. This all-in-one virtual library provides psychiatrists and mental health professionals with key resources for diagnosis, treatment, research, and professional development.
Need more help? PsychiatryOnline Customer Service may be reached by emailing [email protected] or by calling 800-368-5777 (in the U.S.) or 703-907-7322 (outside the U.S.).