Preliminary Investigation of Cerebral Blood Flow and Amyloid Burden in Veterans With and Without Combat-Related Traumatic Brain Injury
Abstract
Methods
TBI Definition
Subjects
Psychiatric Assessment and Apolipoprotein E Status
[15O]Water Imaging and Analysis
[11C]PIB Imaging and Analysis
Statistical Analyses
Results
Background Characteristics and Psychiatric Assessment
Characteristic | Non-TBI Controls (N=11) | TBI Cases (N=8) |
---|---|---|
Demographic information | ||
Age (years) | 30.4 (5.2) | 34.6 (7.8) |
Education (years) | 13.6 (1.6) | 14.5 (2.4) |
Time since trauma or return from deployment (months) | 37.5 (30.2) | 39.0 (11.9) |
Neuropsychological information | ||
Clinician-Administered PTSD Scale total score | 54.1 (26.6) | 48.4 (29.7) |
Hamilton Depression Rating Scale score | 17.0 (8.7) | 16.1 (8.9) |
Alcohol Use Identification Test score | 7.9 (4.6) | 7.3 (5.7) |
Cardiovascular risk information | ||
Smoking (%) | 27.3 | 66.7 |
Diabetes (%) | 0 | 12.5 |
Hypercholesterolemia (%) | 9.1 | 0 |
Systolic blood pressure (mm Hg) | 123.9 (10.3) | 119.0 (6.6) |
Body mass index (kg/m2) | 27.2 (5.4) | 25.6 (3.2) |
TBI information | ||
Clinical severityb | — | Mild (N=5); moderate to severe (N=3) |
gCBF
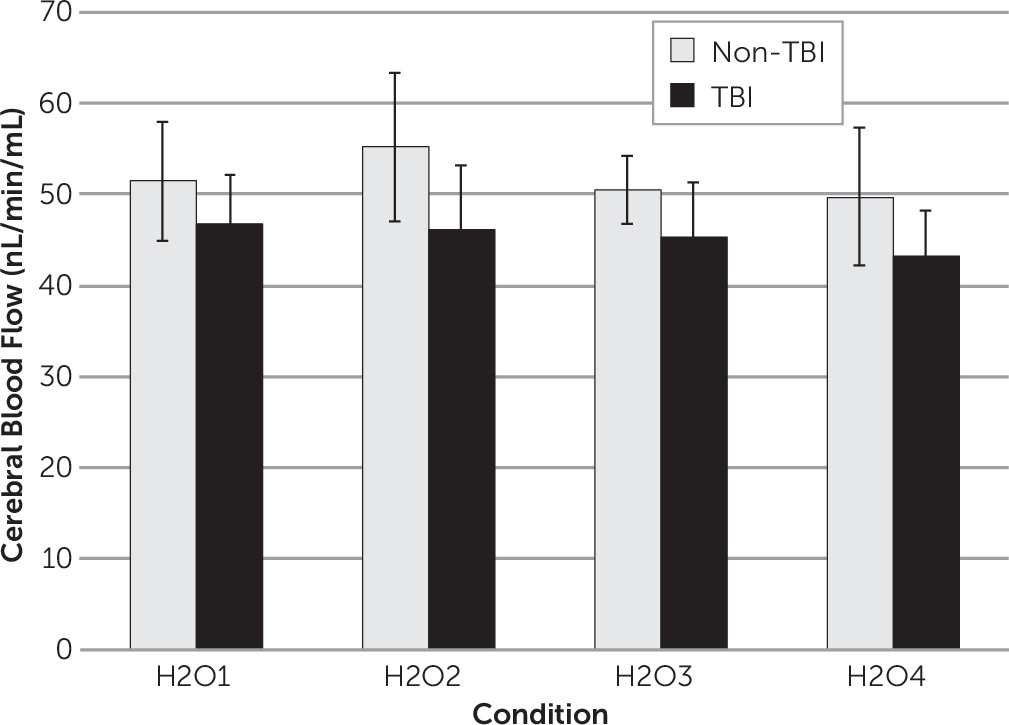
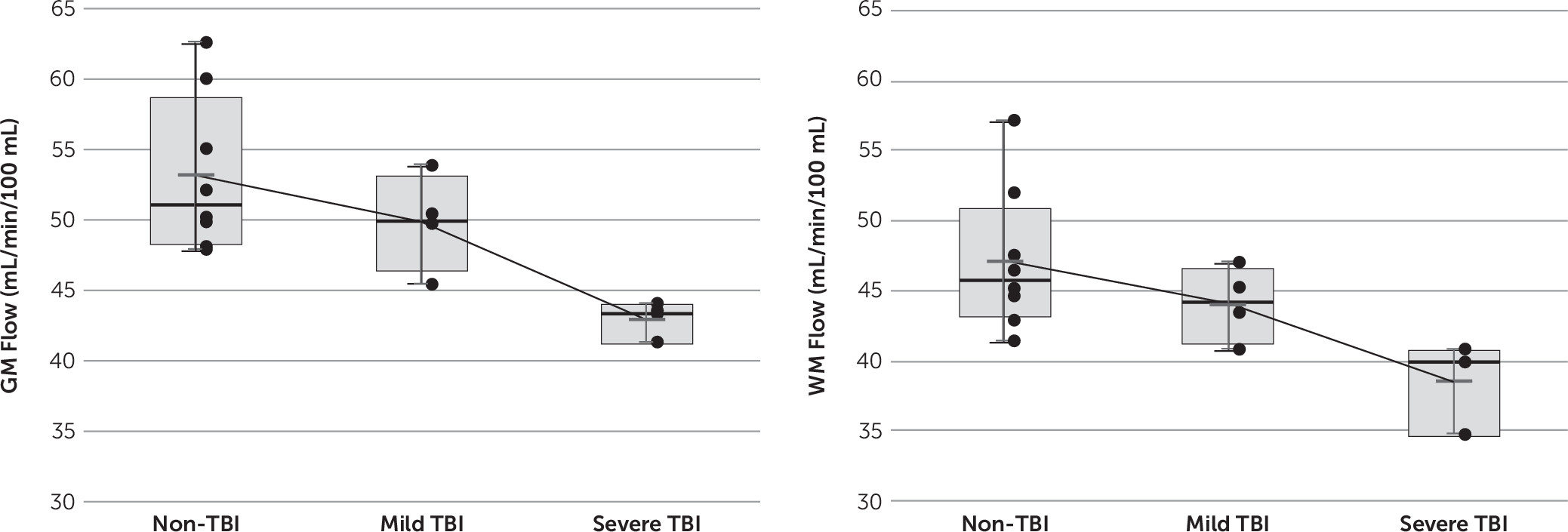
rCBF
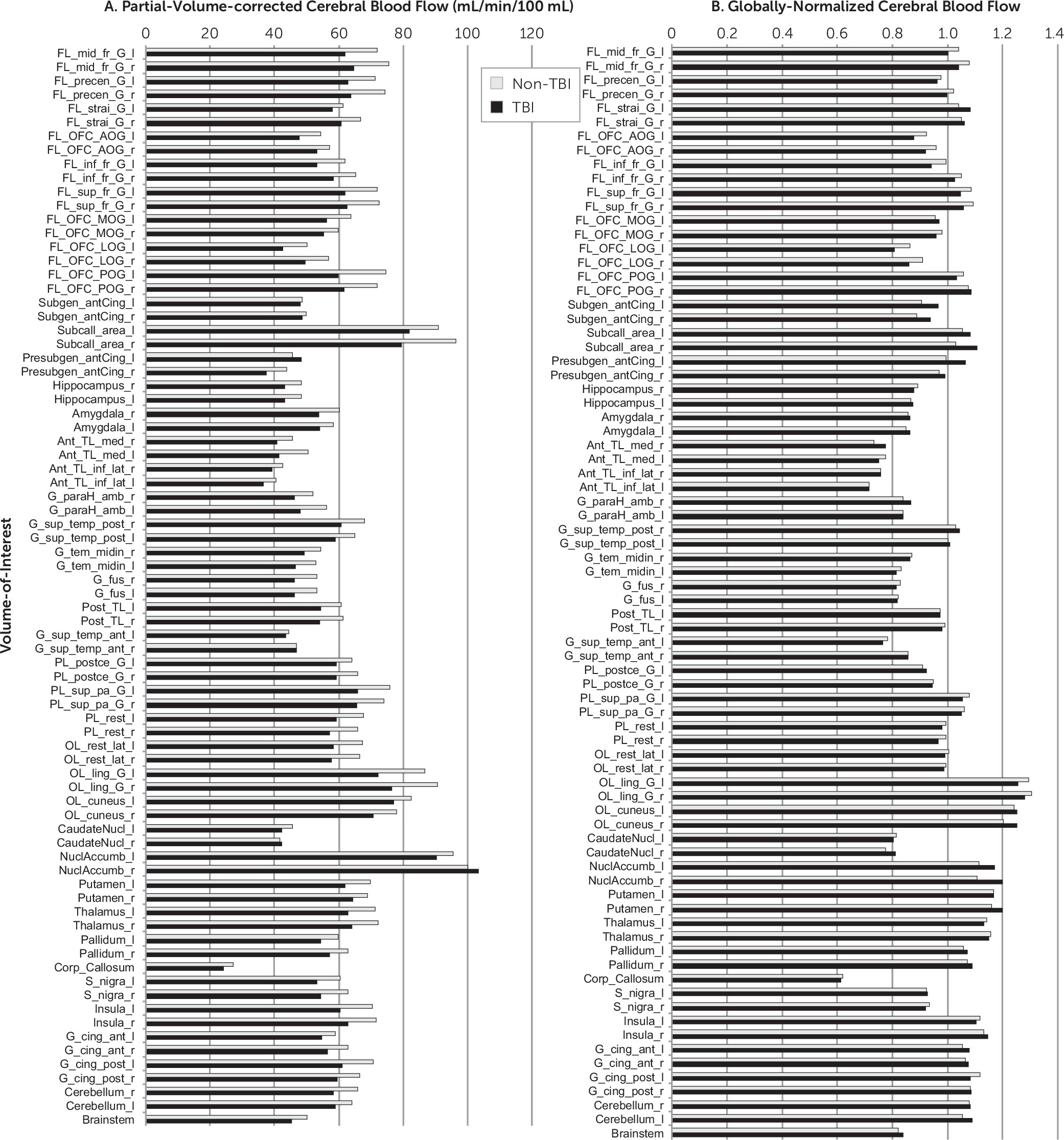
Amyloid Burden
Discussion
Conclusions
Acknowledgments
References
Information & Authors
Information
Published In
History
Authors
Competing Interests
Funding Information
Metrics & Citations
Metrics
Citations
Export Citations
If you have the appropriate software installed, you can download article citation data to the citation manager of your choice. Simply select your manager software from the list below and click Download.
For more information or tips please see 'Downloading to a citation manager' in the Help menu.
View Options
View options
PDF/EPUB
View PDF/EPUBLogin options
Already a subscriber? Access your subscription through your login credentials or your institution for full access to this article.
Personal login Institutional Login Open Athens loginNot a subscriber?
PsychiatryOnline subscription options offer access to the DSM-5-TR® library, books, journals, CME, and patient resources. This all-in-one virtual library provides psychiatrists and mental health professionals with key resources for diagnosis, treatment, research, and professional development.
Need more help? PsychiatryOnline Customer Service may be reached by emailing [email protected] or by calling 800-368-5777 (in the U.S.) or 703-907-7322 (outside the U.S.).