Regional fMRI Hypoactivation and Altered Functional Connectivity During Emotion Processing in Nonmedicated Depressed Patients With Bipolar II Disorder
Abstract
Objective:
Method:
Results:
Conclusions:
Method
Participants
Experimental Stimuli and Paradigm
Whole-Brain fMRI Analysis
Functional Connectivity Analysis
Exploratory Analyses of Clinical Variables and BOLD Response
Results
Participant Data
Characteristic | Depressed Bipolar II Group (N=21) | Healthy Comparison Group (N=21) | Between-Group Differences | ||
---|---|---|---|---|---|
N | % | N | % | pb | |
Female | 10 | 47.6 | 10 | 47.6 | 1.00 |
Race | |||||
Caucasian | 16 | 76.2 | 13 | 61.9 | 0.10 |
African American | 4 | 19.0 | 2 | 9.5 | |
Asian | 1 | 4.8 | 6 | 28.6 | |
Current comorbidity | |||||
Posttraumatic stress disorder | 2 | 9.5 | |||
Anorexia nervosa | 1 | 4.8 | |||
Panic disorder with agoraphobia | 1 | 4.8 | |||
Social phobia | 1 | 4.8 | |||
Past comorbidity | |||||
Social phobia | 1 | 4.8 | |||
Substance use disorders | 7 | 33.3 | |||
Mean | SD | Mean | SD | p | |
Age at scanning (years) | 38.4 | 12.2 | 41.1 | 10.9 | 0.45 |
Inventory of Depressive Symptomatology–Clinician Rated score | 36.4 | 8.7 | |||
Hamilton Depression Rating Scale (HAM-D) | |||||
21-item score | 19.9 | 3.8 | |||
28-item score | 26.7 | 5.2 | |||
Young Mania Rating Scale score | 2.9 | 2.2 | |||
Age at illness onset (years) | 17.8 | 9.0 | |||
Duration of bipolar illness (years) | 21.5 | 11.9 | |||
Duration of current depressive episode (weeks) | 16.7 | 22.5 | |||
Lifetime number of major depressive episodes | 7.1c | 4.6c | |||
Lifetime number of hypomanic episodes | 5.2d | 6.1d | |||
Number of depressive episodes in past 12 months | 2.6 | 1.5 | |||
Number of hypomanic episodes in past 12 months | 3.1 | 3.3 | |||
Lifetime number of hospitalizations for depression | 0.4 | 0.8 | |||
Accuracy (%) | |||||
Match emotions | 84.2 | 9.7 | 87.1 | 17.4 | 0.34 |
Identify emotions | 83.7 | 8.8 | 82.9 | 10.3 | 1.00 |
Match forms (control) | 98.0 | 2.7 | 94.7 | 12.2 | 1.00 |
Reaction time (seconds) | |||||
Match emotions | 2.29 | 0.49 | 2.09 | 0.50 | 0.36 |
Identify emotions | 2.09 | 0.39 | 2.19 | 0.49 | 0.59 |
Match forms (control) | 1.07 | 0.20 | 1.13 | 0.23 | 0.29 |
Behavioral Data
Within-Group Analyses
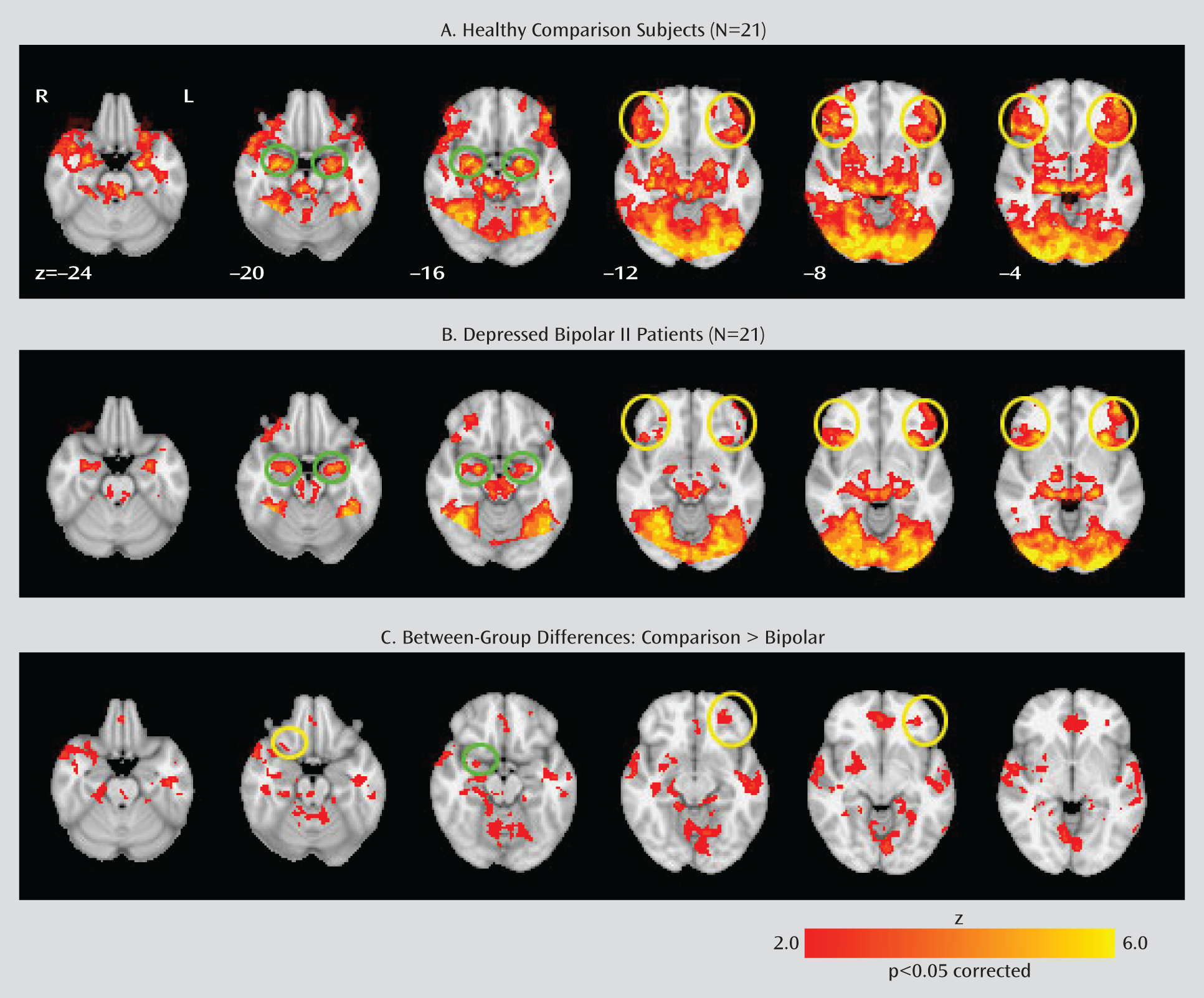
Between-Group Analyses
Region | Brodmann's Area | Montreal Neurological Institute Coordinates (x, y, z)b | z Score (Maximum) |
---|---|---|---|
Frontal lobe | |||
Left inferior frontal gyrus | 47 | –32, 40, –8 | 3.20 |
Right inferior frontal gyrus | 47 | 26, 16, –20 | 2.57 |
Left medial frontal gyrus | 11 | –2, 42, –16 | 3.29 |
Left middle frontal gyrus | 11 | –26, 48, –12 | 2.90 |
Left precentral gyrus | 6 | –42, 0, 40 | 3.40 |
Left insula | 38, –12, 6 | 3.33 | |
Right insula | –36, –14, 16 | 2.27 | |
Cingulate gyrus | |||
Left dorsal anterior cingulate | 32 | –2, 44, –8 | 2.74 |
Right dorsal anterior cingulate | 32 | 2, 44, –8 | 3.17 |
Right ventral anterior cingulate | 24 | –2, 30, 18 | 2.74 |
Subcortical regions | |||
Right amygdala | 26, –2, –16 | 2.42 | |
Left hippocampus | –36, –14, –16 | 2.26 | |
Right hippocampus | 34, –20, –12 | 2.66 | |
Right putamen | 26, 8, –8 | 2.77 | |
Temporal lobe | |||
Left middle temporal gyrus | 21 | –56, –22, –12 | 3.48 |
Right middle temporal gyrus | 21 | 60, –10, –10 | 3.67 |
Left superior temporal gyrus | 22 | 60, –40, 20 | 3.29 |
Right superior temporal gyrus | 22 | 64, –38, 14 | 3.63 |
Right superior temporal gyrus | 39 | 52, –58, 22 | 2.92 |
Parietal lobe | |||
Left inferior parietal lobule | 40 | –52, –52, 40 | 3.64 |
Left supramarginal gyrus | 39 | –50, –54, 32 | 3.24 |
Right supramarginal gyrus | 40 | 60, –52, 24 | 2.60 |
Occipital lobe | |||
Left lingual gyrus | 18 | –4, –82, –8 | 3.53 |
Within-Group Connectivity Analyses
Healthy Comparison Group | Depressed Bipolar II Group | Comparison > Bipolar II | Bipolar II > Comparison | ||||||
---|---|---|---|---|---|---|---|---|---|
Right Amygdala | BA | MNI Coordinates (x, y, z) Peak | Maximum z Score | MNI Coordinates (x, y, z) Peak | Maximum z Score | MNI Coordinates (x, y, z) Peak | Maximum z Score | MNI Coordinates (x, y, z) Peak | Maximum z Score |
Negative connectivity | |||||||||
Left middle frontal gyrus | 46 | –36, 40, 18 | 4.50 | –44, 40, 14 | 3.05 | ||||
Right middle frontal gyrus | 46 | 40, 46, 14 | 5.52 | 40, 36, 28 | 2.74 | 40, 46, 14 | 4.20 | ||
Left middle frontal gyrus | 10 | –24, 54, 8 | 4.81 | –26, 45, –3 | 4.07 | ||||
Right middle frontal gyrus | 10 | 34, 52, –6 | 4.15 | 34, 66, 8 | 3.72 | ||||
Right middle frontal gyrus | 8 | 28, 20, 52 | 3.31 | 28, 26, 46 | 3.40 | ||||
Left superior frontal gyrus | 10 | –20, 52, 16 | 3.75 | –28, 68, 0 | 3.31 | ||||
Right superior frontal gyrus | 10 | 30, 56, 14 | 3.64 | 28, 68, 4 | 3.18 | ||||
Left ventral anterior cingulate cortex | 24 | –2, 28, 20 | 2.85 | –2, 38, –4 | 3.92 | ||||
Right ventral anterior cingulate cortex | 24 | 6, 36, 18 | 3.29 | 4, 38, 4 | 3.79 | ||||
Left dorsal anterior cingulate cortex | 32 | –8, 40, 20 | 2.19 | –2, 38, 30 | 4.66 | ||||
Right dorsal anterior cingulate cortex | 32 | 6, 36, 20 | 3.31 | 2, 42, 18 | 3.63 | ||||
Left inferior parietal lobule | 40 | –40, –58, 44 | 6.25 | –40, –56, 42 | 5.19 | ||||
Right inferior parietal lobule | 40 | 44, –58, 52, | 5.57 | 52, –66, 42 | 4.71 | 54, –44, 52 | 3.78 | ||
Right superior parietal lobule | 7 | 36, –70, 52 | 4.05 | 34, –72, 54 | 3.10 | 26, –68, 48 | 2.69 | ||
Left precuneus | 7 | –6, –66, 46 | 4.25 | –12, –66, 46 | 4.86 | ||||
Right precuneus | 7 | 8, –74, 40 | 4.73 | 2, –78, 52 | 4.70 | ||||
Left cuneus | 18/19 | –8, –76, 20 | 5.26 | –12, –76, 34 | 3.77 | ||||
Right cuneus | 18/19 | 8, –72, 14 | 5.26 | 8, –74, 32 | 4.67 | ||||
Positive connectivity | |||||||||
Left inferior frontal gyrus | 47 | –28, 15, –15 | 2.99 | –18, 14, –22 | 3.06 | –22, 16, –22 | 2.12 | ||
Left insula | –42, –4, –8 | 3.23 | –42, –6, –8 | 3.88 | –34, 2, 10 | 3.17 | |||
Right precentral gyrus | 4 | 60, 2, 6 | 3.13 | 52, –2, 12 | 2.72 | ||||
Right postcentral gyrus | 43 | 56, –12, 14 | 3.23 | 56, –10, 14 | 3.55 | ||||
Right superior temporal | 38 | 42, 20, –26 | 6.16 | 40, 18, –26 | 6.69 | ||||
Right superior temporal | 22 | 50, –10, –4 | 2.47 | 62, –2, 4 | 3.04 | 66, –4, 4 | 2.97 | ||
Left putamen | –24, 2, –6 | 3.28 | –26, 4, –10 | 4.78 | –30, –10, –2 | 3.01 | |||
Left amygdala | –24, –6, –16 | 6.47 | –28, –2, –24 | 6.51 | –28, –6, –20 | 2.04 | |||
Right amygdala | 26, –4, –18 | 9.95 | 22, –6, –16 | 10.00 | |||||
Left hippocampus | –24, –6, –18 | 6.38 | –28, –10, –18 | 7.18 | –28, –8, –20 | 2.98 |
Between-Group Connectivity Analyses
Negative functional connectivity.
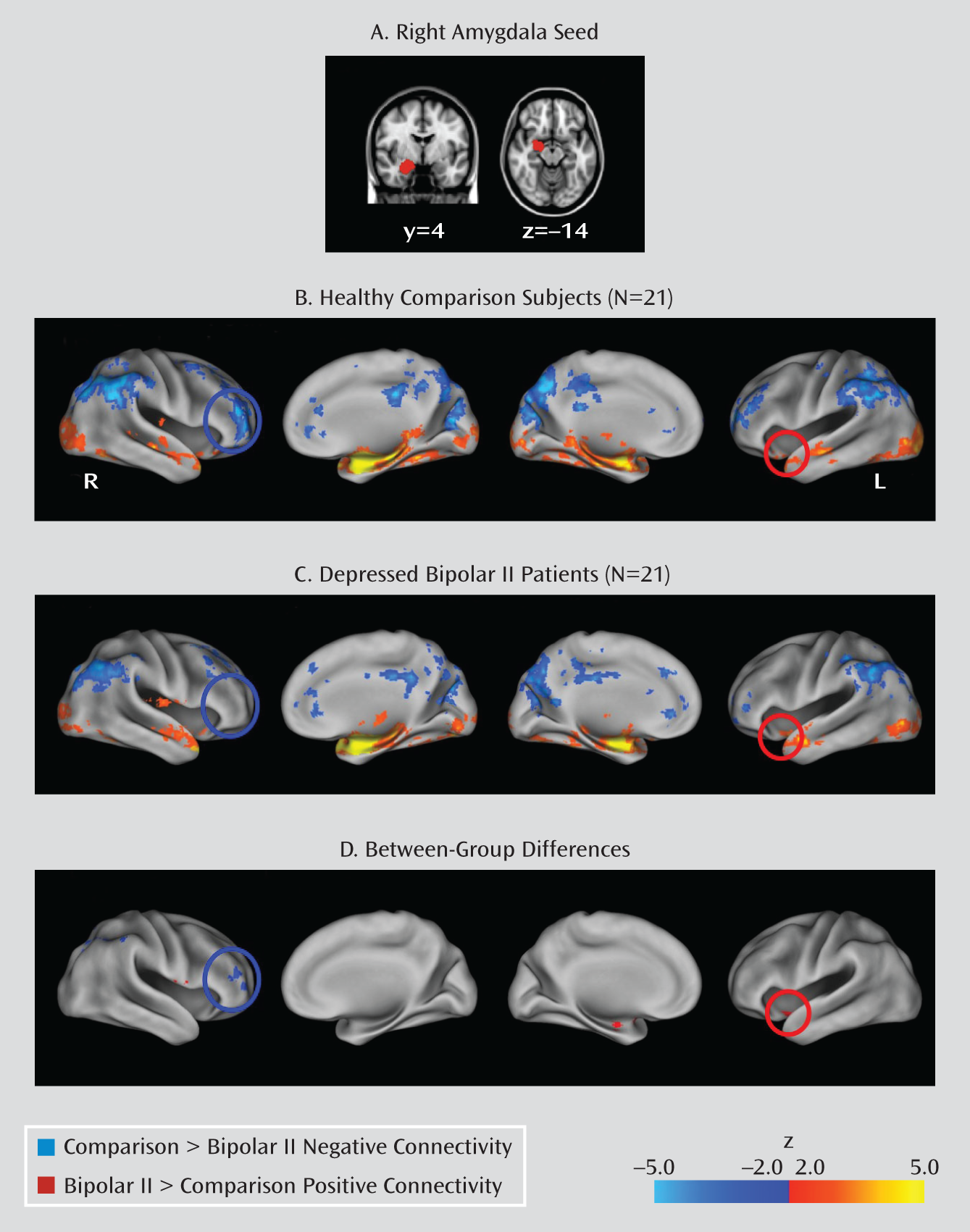
Positive functional connectivity.
Exploratory Analyses Examining Relationships Between Clinical Variables and BOLD Response
Discussion
Acknowledgments
Footnotes
Supplementary Material
- View/Download
- 27.30 KB
References
Information & Authors
Information
Published In
History
Authors
Funding Information
Metrics & Citations
Metrics
Citations
Export Citations
If you have the appropriate software installed, you can download article citation data to the citation manager of your choice. Simply select your manager software from the list below and click Download.
For more information or tips please see 'Downloading to a citation manager' in the Help menu.
View Options
View options
PDF/EPUB
View PDF/EPUBLogin options
Already a subscriber? Access your subscription through your login credentials or your institution for full access to this article.
Personal login Institutional Login Open Athens loginNot a subscriber?
PsychiatryOnline subscription options offer access to the DSM-5-TR® library, books, journals, CME, and patient resources. This all-in-one virtual library provides psychiatrists and mental health professionals with key resources for diagnosis, treatment, research, and professional development.
Need more help? PsychiatryOnline Customer Service may be reached by emailing [email protected] or by calling 800-368-5777 (in the U.S.) or 703-907-7322 (outside the U.S.).