A Genome Scan for Loci Shared by Autism Spectrum Disorder and Language Impairment
Abstract
Objective
Method
Results
Conclusion
Method
Overview of Design
Genotyping
Statistical Analysis
Overall data analysis plan.
Linkage/association analysis methods.
Statistical correction for multiple phenotypes.
Results
Initial Linkage Results for Language
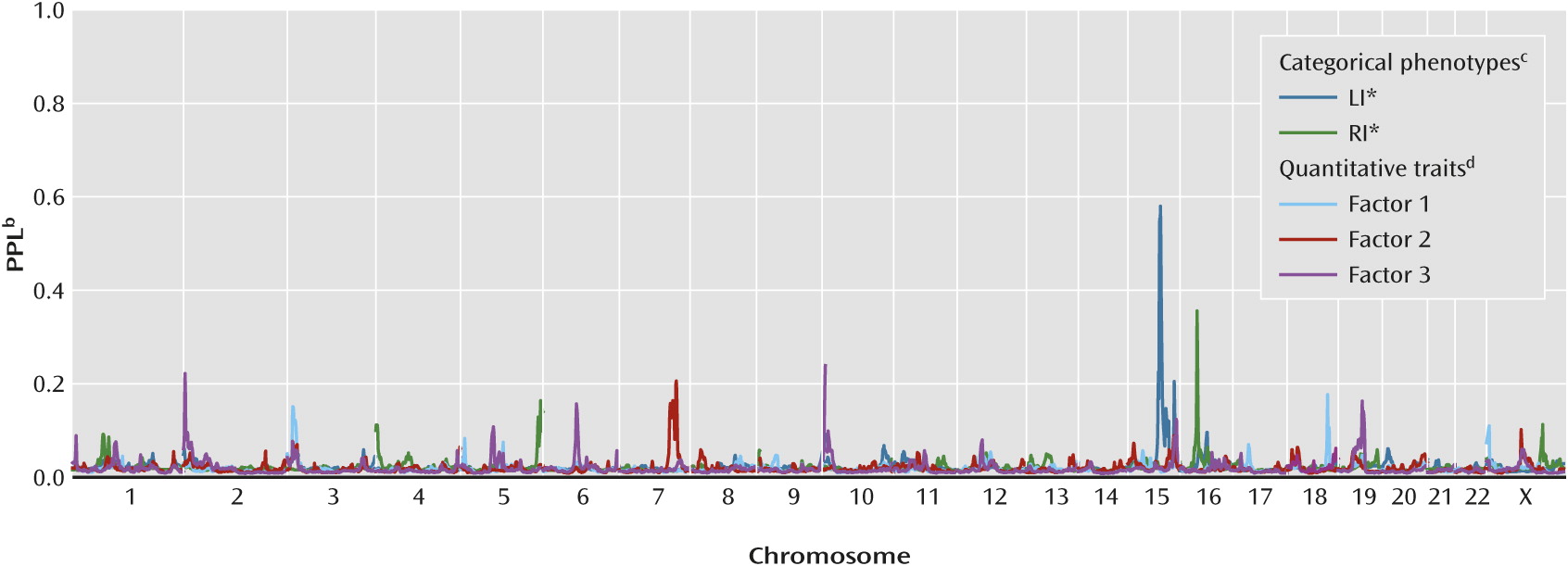
Maximizing Model | |||||||||||
---|---|---|---|---|---|---|---|---|---|---|---|
Estimated Genotypic Effect for Locusc | |||||||||||
Phenotype | Chromosome | cM | PPL | Band Range | Width (Mb) | Fully Maximized Lod Scoreb | −/− | +/− | +/+ | Disease Gene Frequency | Heterogeneity Parameter in Admixture Likelihood (α)d |
Yale-Brown Obsessive Compulsive Scale | 13 | 58 | 0.36 | q14.3–21.3 | 17.3 | 4.2 | –3.0 | 0.00 | 3.00 | 0.300 | 0.7 |
Social Responsiveness Scale dichotomous trait | 14 | 117 | 0.37 | q32.2–32.23 | 7.6 | 3.5 | 0.0 | 0.10 | 0.80 | 0.100 | 1.0 |
LI* traite | 15 | 83 | 0.57 | q23–26.2 | 24.2 | 4.1 | 0.0 | 0.70 | 0.99 | 0.001 | 1.0 |
Social Responsiveness Scale quantitative trait | 15 | 120 | 0.52 | q26.2–26.3 | 6.2 | 4.5 | –2.0 | 1.00 | 2.00 | 0.100 | 0.9 |
RI* traite | 16 | 43 | 0.36 | p12.1–12.3 | 8.9 | 4.6 | 0.0 | 0.00 | 0.90 | 0.200 | 1.0 |
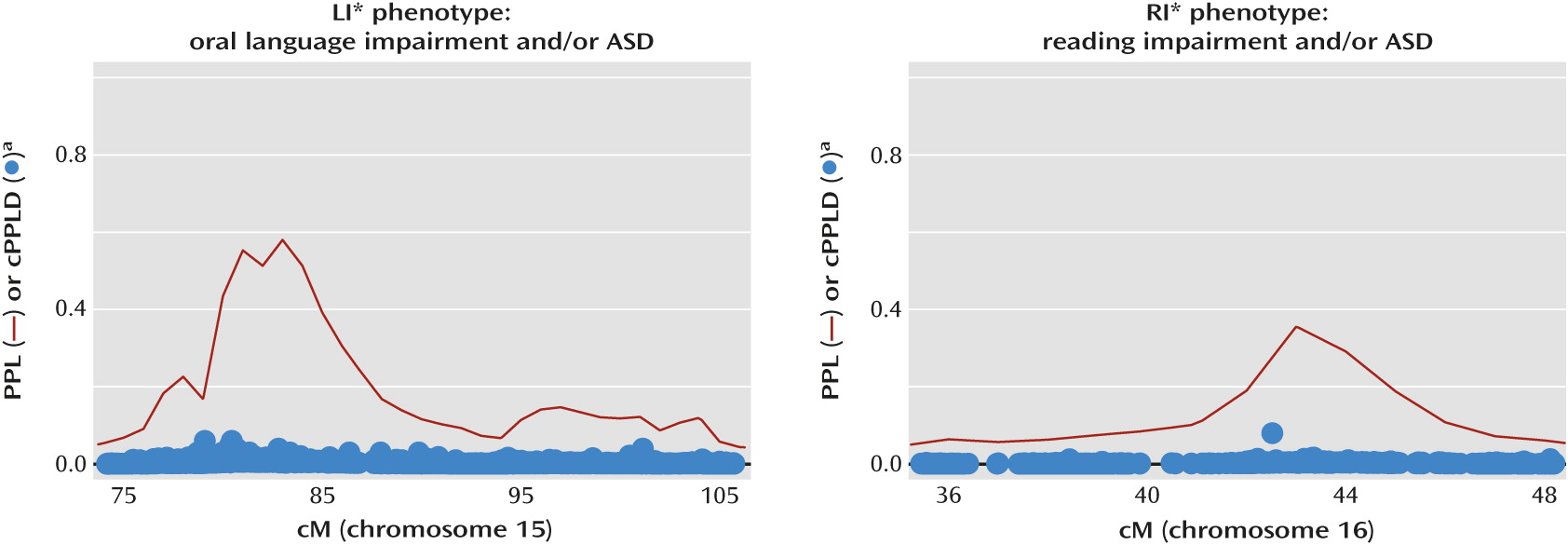
Further Characterization of the Roles of Language and Autism at Linked Loci
Linkage Analysis of Nonlanguage Phenotypes
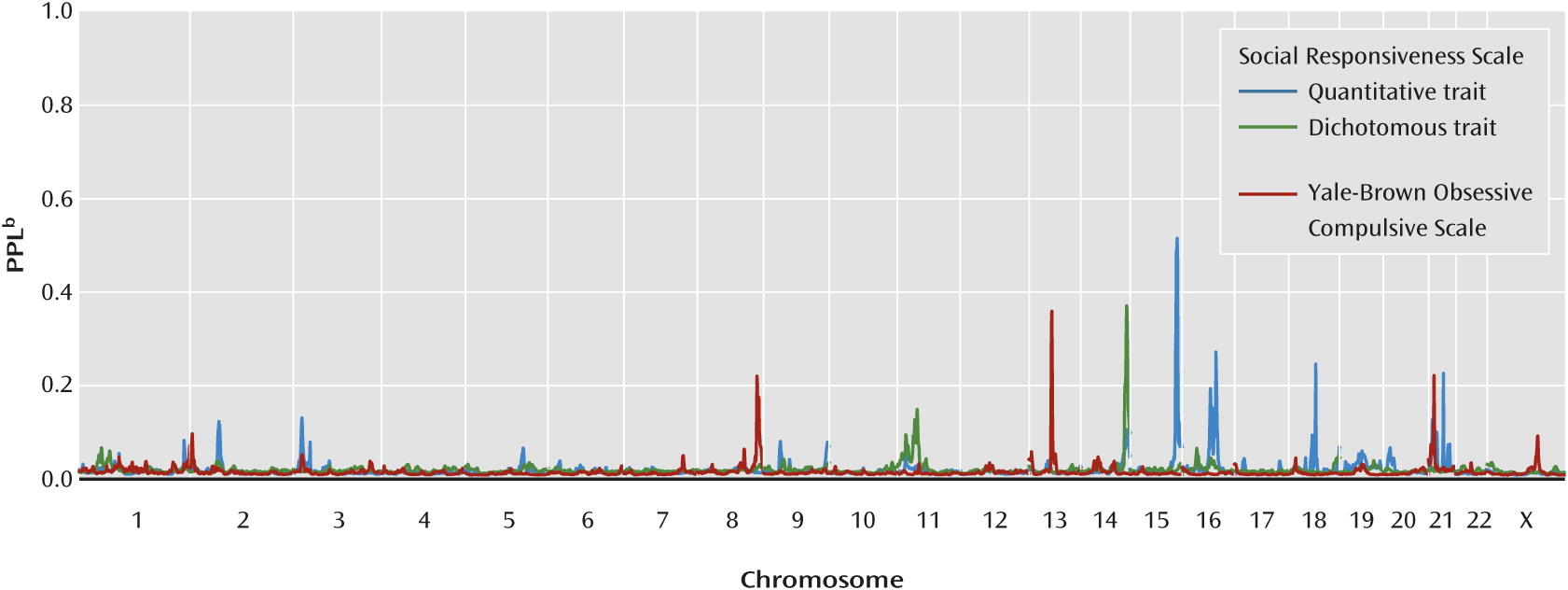
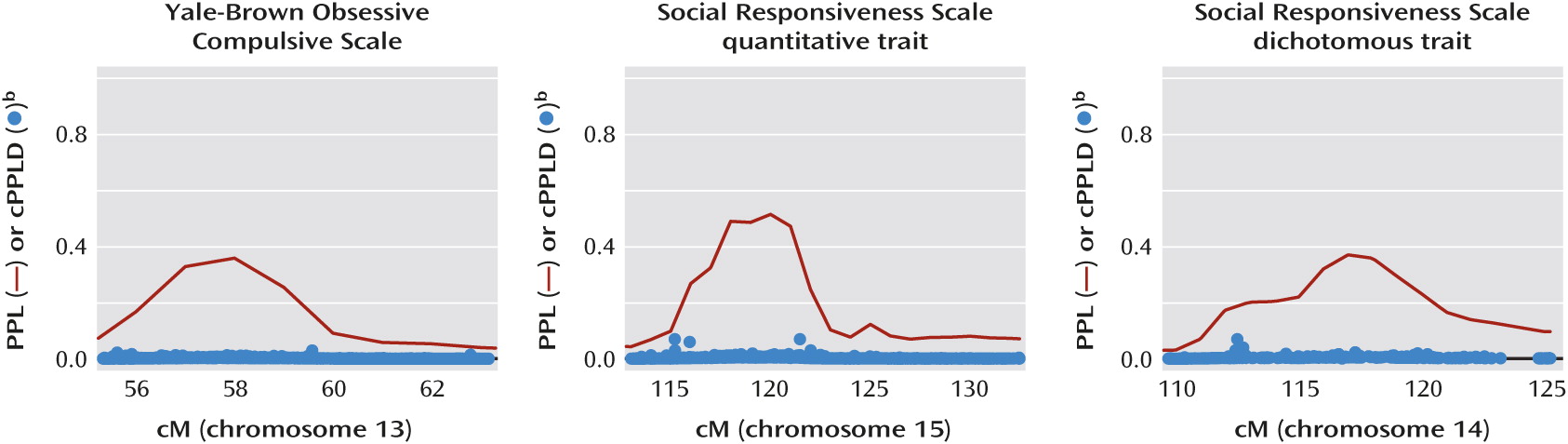
Genome-Wide Association Analysis
Discussion
Acknowledgments
Supplementary Material
- View/Download
- 326.52 KB
References
Information & Authors
Information
Published In
History
Authors
Funding Information
Metrics & Citations
Metrics
Citations
Export Citations
If you have the appropriate software installed, you can download article citation data to the citation manager of your choice. Simply select your manager software from the list below and click Download.
For more information or tips please see 'Downloading to a citation manager' in the Help menu.
View Options
View options
PDF/EPUB
View PDF/EPUBLogin options
Already a subscriber? Access your subscription through your login credentials or your institution for full access to this article.
Personal login Institutional Login Open Athens loginNot a subscriber?
PsychiatryOnline subscription options offer access to the DSM-5-TR® library, books, journals, CME, and patient resources. This all-in-one virtual library provides psychiatrists and mental health professionals with key resources for diagnosis, treatment, research, and professional development.
Need more help? PsychiatryOnline Customer Service may be reached by emailing [email protected] or by calling 800-368-5777 (in the U.S.) or 703-907-7322 (outside the U.S.).