The Overlapping Neurobiology of Induced and Pathological Anxiety: A Meta-Analysis of Functional Neural Activation
Abstract
Objective:
Methods:
Results:
Conclusions:
Methods
Literature Search and Article Inclusion
SDM Meta-Analysis Procedure
Results
Pathological Anxiety–Associated Brain Activity
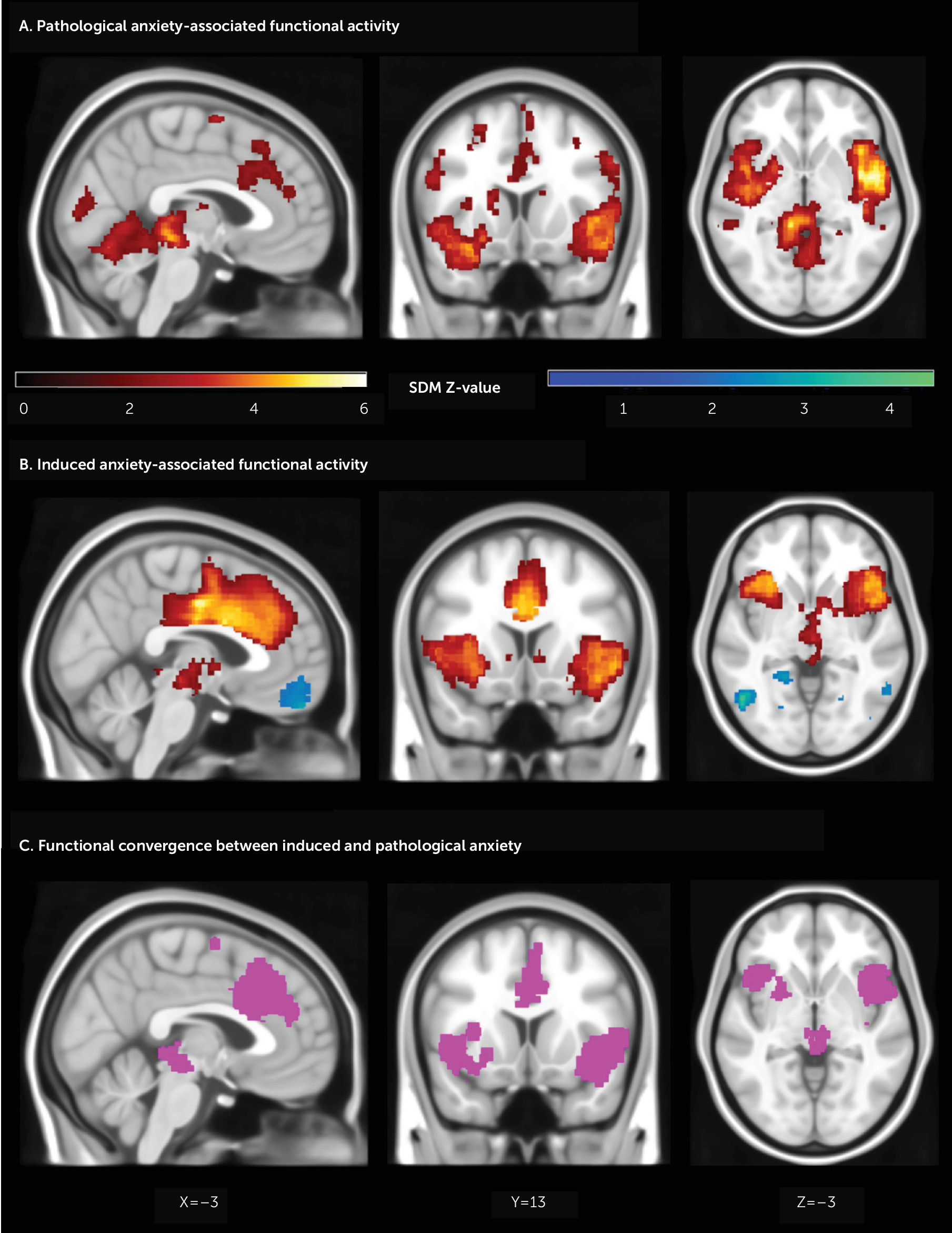
MNI Coordinates (x, y, z) | Voxels | Z | Description | Egger’s Intercept | Egger’s p |
---|---|---|---|---|---|
Pathological anxiety | |||||
–36, 6, –14 | 12,836 | 5.413 | L. insula, inferior frontal gyrus (all), putamen, pallidum, Rolandic operculum, precentral gyrus, postcentral gyrus, middle frontal gyrus, Heschl’s gyrus, superior temporal gyrus pole, superior temporal gyrus, middle temporal gyrus pole, middle temporal gyrus, amygdala, hippocampus, L. and R. parahippocampal gyrus, L. and R. vermis 3–7, L. and R. cerebellum 3–6, cerebellum 8, L. and R. lingual gyrus, L. and R. fusiform gyrus, L. and R. thalamus | 0.34 | 0.519 |
48, 4, –14 | 5,701 | 6.159 | R. insula, inferior frontal gyrus (all), superior temporal gyrus pole, superior temporal gyrus, middle temporal gyrus pole, middle temporal gyrus, Heschl’s gyrus, Rolandic operculum, inferior temporal gyrus | 0.46 | 0.355 |
–10, –2, 68 | 1,203 | 3.951 | L. and R. midcingulate cortex, supplementary motor area, medial frontal gyrus, anterior cingulate cortex, paracentral lobule | 0.25 | 0.666 |
–36, –74, 30 | 565 | 3.589 | L. middle occipital gyrus, inferior parietal gyrus, superior occipital gyrus, superior parietal gyrus | –0.17 | 0.722 |
4, –90, 12 | 333 | 3.362 | Center of calcarine, L. and R. cuneus | 0.39 | 0.397 |
–26, 22, 40 | 280 | 3.149 | L. middle frontal gyrus, superior frontal gyrus | 0.22 | 0.698 |
–16, 12, 14 | 202 | 2.975 | L. caudate, thalamus | 0.09 | 0.863 |
18, –56, 50 | 180 | 2.667 | R. superior parietal gyrus, precuneus, inferior parietal gyrus | 0.09 | 0.859 |
20, –76, 34 | 130 | 2.992 | R. superior occipital gyrus, cuneus | 0.11 | 0.828 |
22, –16, –26 | 112 | 3.146 | R. parahippocampal gyrus, hippocampus | 0.20 | 0.685 |
–14, –58, 58 | 64 | 3.034 | L. precuneus, superior parietal gyrus | 0.26 | 0.611 |
8, –66, 18 | 65 | 2.574 | R. calcarine, cuneus | 0.01 | 0.992 |
14, 10, 18 | 41 | 2.536 | R. caudate | 0.17 | 0.737 |
–44, –20, 56 | 37 | 2.557 | L. postcentral gyrus | 0.14 | 0.790 |
34, 12, 44 | 30 | 2.279 | R. middle frontal gyrus | 0.03 | 0.948 |
58, –32, 40 | 22 | 2.308 | R. supramarginal gyrus | 0.08 | 0.875 |
10, –2, 66 | 21 | 2.433 | R. supplementary motor area | 0.05 | 0.916 |
12, –28, 40 | 14 | 2.201 | R. midcingulate cortex | 0.14 | 0.780 |
–8, 6, 38 | 10 | 2.114 | L. midcingulate cortex | 0.05 | 0.927 |
Induced anxiety | |||||
4, 38, 38 | 6,538 | 6.415 | Anterior cingulate cortex, midcingulate cortex, superior medial frontal gyrus | 1.48 | 0.110 |
50, 22, 2 | 4,537 | 5.183 | R. insula, inferior frontal gyrus (all), Rolandic operculum, superior temporal gyrus pole | 1.91 | 0.148 |
–30, 18, –14 | 1,811 | 5.067 | L. insula, inferior frontal gyrus (all), putamen, Rolandic operculum | 1.30 | 0.199 |
60, –46, 36 | 1,373 | 4.458 | R. supramarginal gyrus, superior temporal gyrus, angular gyrus | 0.78 | 0.553 |
46, 2, 48 | 336 | 3.590 | R. precentral gyrus, middle frontal gyrus | 1.53 | 0.161 |
–56, –44, 28 | 303 | 3.354 | L. supramarginal gyrus | 1.28 | 0.199 |
35, 52, 18 | 153 | 2.500 | R. middle frontal gyrus, superior frontal gyrus | 2.65 | 0.222 |
–10, –34, –48 | 36 | 3.019 | Possible cerebellum 9, 10 | 0.30 | 0.778 |
–48, –62, –6 | 1,251 | –4.117 | L. inferior temporal gyrus, middle temporal gyrus, inferior occipital gyrus | –0.32 | 0.752 |
–56, –22, 46 | 636 | –4.492 | L. postcentral gyrus, inferior parietal gyrus | –0.38 | 0.726 |
54, –60, –10 | 559 | –4.102 | R. inferior temporal gyrus, inferior occipital gyrus, middle temporal gyrus, middle occipital gyrus | –0.14 | 0.891 |
–8, 52, –22 | 478 | –3.267 | L. orbital medial frontal gyrus | –0.11 | 0.932 |
42, –44, –16 | 188 | –3.391 | R. fusiform gyrus | –0.25 | 0.826 |
–12, –64, 12 | 185 | –3.132 | L. calcarine, lingual gyrus | –0.12 | 0.912 |
–20, –46, 0 | 121 | –3.063 | L. lingual gyrus, fusiform gyrus | –0.32 | 0.769 |
26, –62, –8, | 47 | –2.525 | R. fusiform gyrus, lingual gyrus | –0.34 | 0.744 |
–20, –70, –8 | 44 | –2.697 | L. fusiform gyrus, lingual gyrus | –0.38 | 0.728 |
–32, –26, –20 | 42 | –2.675 | L. fusiform gyrus, parahippocampal gyrus | –0.42 | 0.695 |
12, –70, 16 | 41 | –2.691 | R. calcarine | –0.09 | 0.940 |
–22, –16, –22 | 37 | –3.052 | L. parahippocampal gyrus, hippocampus | –0.62 | 0.608 |
28, –24, –24 | 13 | –2.448 | R. parahippocampal gyrus, fusiform gyrus | 0.08 | 0.945 |
18, –78, 14 | 12 | –2.119 | R. calcarine | –0.21 | 0.842 |
Convergence | |||||
–12, –8, 68 | 2,217 | Supplementary motor area, midcingulate cortex, medial frontal gyrus (superior), anterior cingulate cortex | |||
44, 14, –14 | 2,102 | R. insula, inferior frontal gyrus (all), superior temporal gyrus pole, Rolandic operculum, superior temporal gyrus, putamen | |||
–38, 20, –8 | 1,305 | L. insula, inferior frontal gyrus (all), putamen, Rolandic operculum, superior temporal gyrus pole | |||
–4, –22, –10 | 615 | R. thalamus, vermis 3 | |||
50, 2, 44 | 183 | R. precentral gyrus, middle frontal gyrus | |||
12, –26, 40 | 43 | R. midcingulate cortex |
Diagnostic Group Analyses
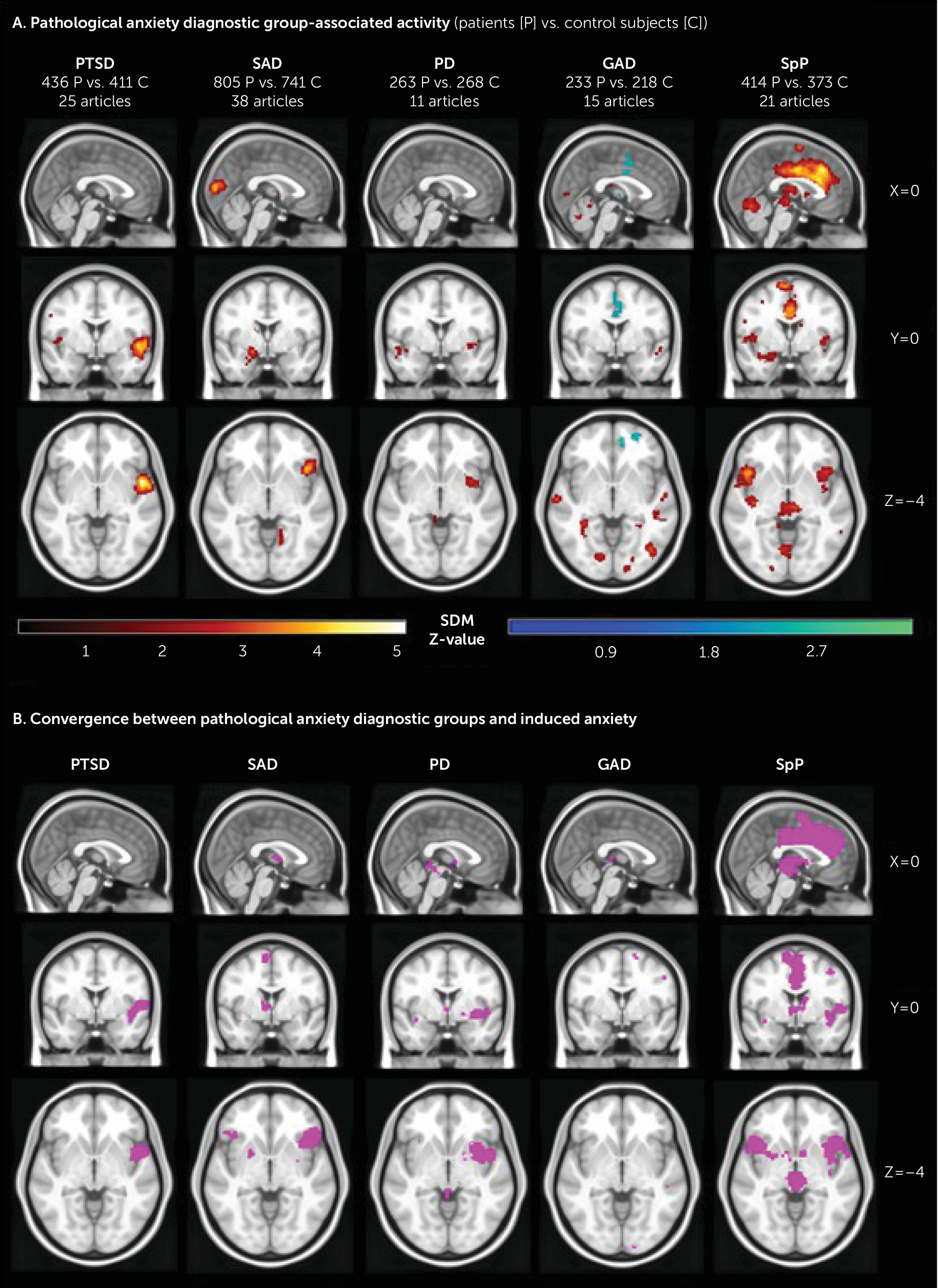
Induced Anxiety–Associated Brain Activity
Comparison Between Induced and Pathological Anxiety
Diagnostic group analyses.
Overlap of Induced Anxiety With Fear Conditioning
Discussion
Induced Anxiety as a Model for Pathological Anxiety
Specificity Across Disorders
Limitations
Supplementary Material
- View/Download
- 737.64 KB
References
Information & Authors
Information
Published In
History
Keywords
Authors
Competing Interests
Metrics & Citations
Metrics
Citations
Export Citations
If you have the appropriate software installed, you can download article citation data to the citation manager of your choice. Simply select your manager software from the list below and click Download.
For more information or tips please see 'Downloading to a citation manager' in the Help menu.
View Options
View options
PDF/EPUB
View PDF/EPUBGet Access
Login options
Already a subscriber? Access your subscription through your login credentials or your institution for full access to this article.
Personal login Institutional Login Open Athens loginNot a subscriber?
PsychiatryOnline subscription options offer access to the DSM-5-TR® library, books, journals, CME, and patient resources. This all-in-one virtual library provides psychiatrists and mental health professionals with key resources for diagnosis, treatment, research, and professional development.
Need more help? PsychiatryOnline Customer Service may be reached by emailing [email protected] or by calling 800-368-5777 (in the U.S.) or 703-907-7322 (outside the U.S.).