Learning About Safety: Conditioned Inhibition as a Novel Approach to Fear Reduction Targeting the Developing Brain
Abstract
Fear Learning and Interventions for Anxiety Disorders
Term | Definition |
---|---|
Fear learning | An adaptive function that allows an organism to predict potentially aversive events from environmental cues. |
Unconditioned stimulus, US | A stimulus that reliably produces a natural, or unconditioned, response. In fear conditioning, this stimulus is aversive in nature (e.g., shock, aversive noise). |
Conditioned stimulus, CS | A previously neutral stimulus that is paired with a US in classical conditioning (acquisition phase). |
Fear extinction | The gradual process of fear reduction in classical conditioning that involves repeated exposure to the CS without the US. |
Safety learning | The process of learning about safety in the environment. Tested using threat and safety discrimination. |
Safety signal learning | The process through which a stimulus that is overly trained to signal the absence of threat (i.e., the safety cue) reduces fear in the presence of a threatening cue. Tested using the summation and retardation tests. |
Conditioned inhibitor | A stimulus that inhibits a response as a result of learning (i.e., acquisition). |
External inhibitor | A stimulus that inhibits a response without having undergone explicit training. |
Safety behavior | An action or behavior that involves the use of a safety cue. |
Preventive safety behavior | An action that employs a safety behavior prior to an anticipated threatening event to avoid harm or reduce the intensity of the event. |
Restorative safety behavior | An action that employs a safety behavior following an aversive event with the goal of restoring safety. |
Neural Mechanisms of Fear Reduction
Harnessing Translational Research to Optimize Treatments
Safety Signal Learning as an Approach for Fear Reduction
Safety Signal Learning | Extinction Learning | |
---|---|---|
Definition | The process through which a stimulus that is overly trained to signal the absence of threat (i.e., the safety cue) reduces, or inhibits, fear in the presence of a threatening cue. Safety signal learning is a class of conditioned inhibition. | The gradual process of fear reduction in classical conditioning that involves repeated exposure to the conditioned stimulus (CS) without the unconditioned stimulus (US). |
Key neural mechanisms | Ventral hippocampal connections with the prelimbic cortex in rodents and the dACC in humans are hypothesized to be involved in inhibiting fear in the presence of safety (107). Dopamine receptors in the basolateral amygdala may also be involved in safety signal learning (208). | The amygdala, vmPFC, and hippocampus are central to fear learning and extinction (23, 31–34). The infralimbic cortex in rodents and the anterior vmPFC in humans inhibit fear expression and store and retrieve the extinction memory (54, 113). |
Age-related differences | Developmental studies are lacking. Hypothesized age-related differences such that safety signal learning may be augmented in adolescence. | Diminished fear extinction learning has been observed during adolescence across species (28, 29). |
Limitations | Safety cues may prevent the generalization of inhibitory learning (146, 153, 159, 160, 162), communicate threat, increase perception of threat, and direct attention away from information that is disconfirmatory during exposures (for a review, see 146). | Susceptible to relapse of extinguished fear (extinction memory does not overwrite the original fear association) via the mere passage of time (spontaneous recovery), exposure to a stressor (fear reinstatement), or return to a fear-associated context (fear renewal) (for a review, see 209). |
Neural Mechanisms of Safety Signal Learning via Conditioned Inhibition
Authors, Year, Reference | Sample | Region of Interest | Method | Results |
---|---|---|---|---|
Kazama et al. 2012 (120) | Nonhuman primates | Amygdala | Neonatal neurotoxic lesion | Delayed, but not abolished, acquisition of fear; no effect on summation test |
Falls and Davis 1995 (121) | Rodents | CeA | Bilateral electrolytic lesion | No interference with acquisition of conditioned fear inhibition by safety cues |
Sangha et al. 2013 (119) | Rodents | BLA | In vivo single unit recordings | Subpopulation of neurons in the BLA selectively responsive to safety cues |
Ng et al. 2018 (208) | Rodents | BLA | Altering dopamine (D1) receptors | D1 receptor alterations (agonist and antagonist) experimentally impair suppression of fear during a safety cue |
Sangha et al. 2014 (125) | Rodents | vmPFC (PL and IL separately) | Inactivation using muscimol/baclofen mixture | Disrupted summation test following IL inactivation |
Rhodes and Killcross 2007 (126) | Rodents | vmPFC (IL) | Lesion | No effect on summation test, but disrupted retardation |
Gewirtz et al. 1997 (127) | Rodents | Large medial PFC region | Lesion | No effect on safety learning, extinction, or retardation |
Kazama et al. 2014 (128) | Nonhuman primates | OFC | Neonatal neurotoxic lesions | No deficits in modulating fear responses in the presence of safety signals |
Sarlitto et al. 2018 (138) | Rodents | vlOFC | Inactivation using muscimol/baclofen mixture | No effect on discrimination if inactivated during acquisition; inactivation after acquisition and prior to recall impaired discrimination, with the behavioral fear response prevailing |
Fernando et al. 2014 (139) | Rodents | NAc shell | Infusion of dextroamphetamine | Reduced inhibition of avoidance behavior |
Josselyn et al. 2005 (134) | Rodents | NAc | Electrolytic lesion | No impact on acquisition or expression of conditioned inhibition during fear-potentiated startle |
Rogan et al. 2005 (135) | Rodents | Striatum | Electrophysiological recording | Enhanced responses in safety but weakened in fear conditioning, suggesting that safety cues may increase approach behavior |
Christianson et al. 2008 (136) | Rodents | Posterior insula | Excitotoxic lesion | Blocked inhibition via safety cues if lesioned prior to acquisition |
Christianson et al. 2011 (137) | Rodents | Posterior insula | Inactivation using muscimol | Abolished stress-mitigating effects of safety signal learning, but not behavioral control, if lesioned during exposure to uncontrollable stress |
Heldt et al. 2002 (115) | Rodents | Hippocampus | Aspiration lesion | Pretraining lesion did not impair inhibition of conditioned responses, but posttraining lesion did impair performance |
Chan et al. 2003 (116) | Rodents | Hippocampus | Ibotenate lesion | No effect on summation test, though authors note marginal difference such that lesion group responded more to stimulus compound than control group |
Pollak et al. 2008 (117) | Rodents | Hippocampus | Immunohistochemistry and X-irradiation treatment | Increased neurogenesis following safety signal learning; inhibiting neurogenesis prevented the behavioral effects of safety signal learning |
McDonald et al. 2018 (118) | Rodents | Ventral hippocampus | Neurotoxic lesion | Impaired expression of conditioned inhibition such that the lesion group acquired the reversal task more efficiently than the control group |
Meyer et al. 2019 (107) | Rodents and humans | Ventral hippocampus | Fiber photometry and fMRI | Summation test passed in both humans and mice; VH activation related to conditioned inhibition in both humans and mice; activation of VH neurons projecting to PL associated with conditioned inhibition and correlated with freezing in rodents; functional connectivity of VH-dACC associated with conditioned inhibition in humans |
Clinical Translation: Safety Cues in Therapy and the Real World
Mechanisms by Which Safety Cues May Enhance Or Interfere With Treatment Outcomes
Enhancing treatment acceptability.
Facilitating approach behavior.
Enhancing inhibitory learning.
Interfering with inhibitory learning.
Conditions Under Which Safety Cues May Enhance Or Interfere With Treatment
Type of safety cue.
Developmental stage.
Proposed Theoretical Model of Clinical and Neurobiological Mechanisms by Which Safety Signal Learning Reduces Fear
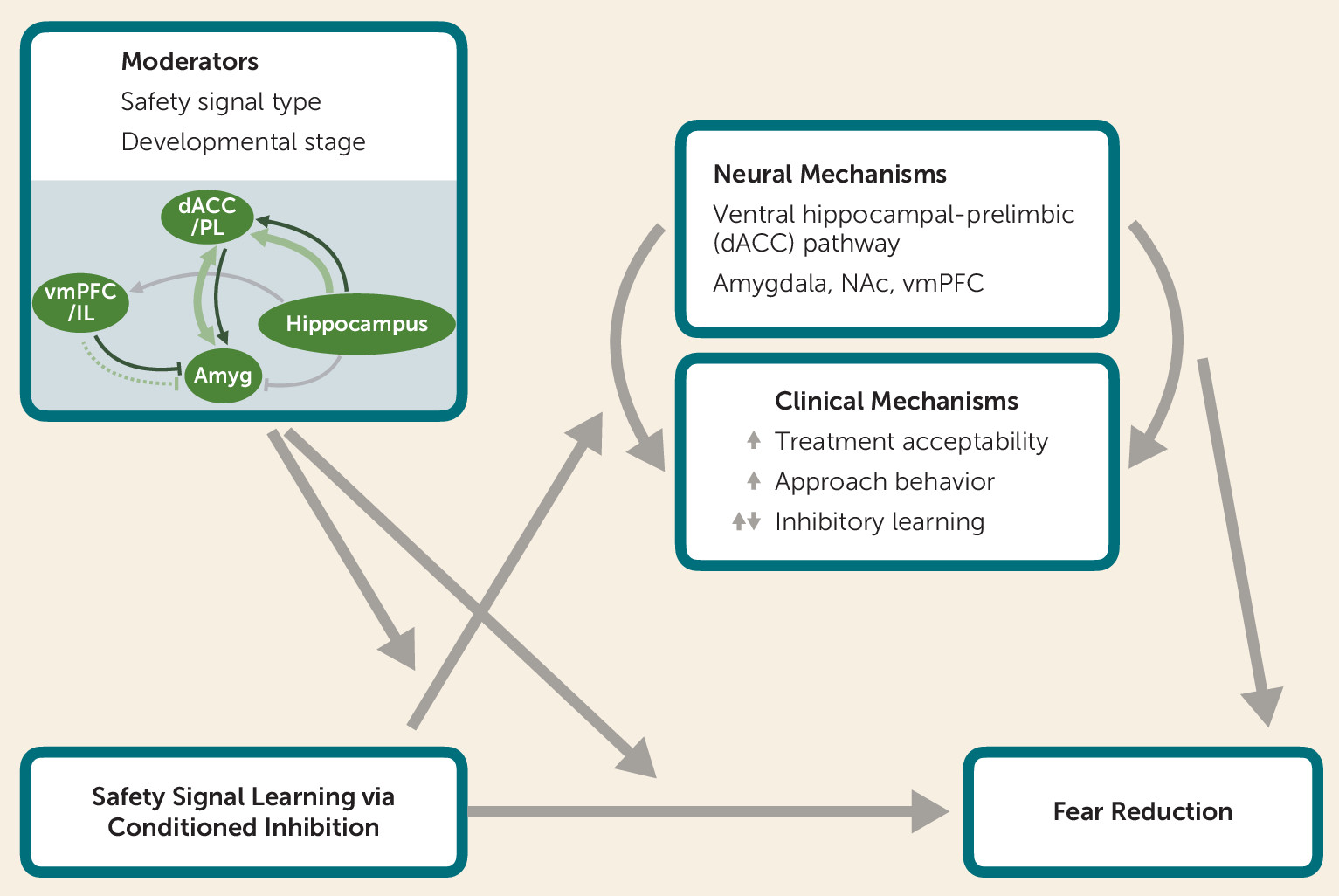
Safety Signal Learning Across Neurodevelopment
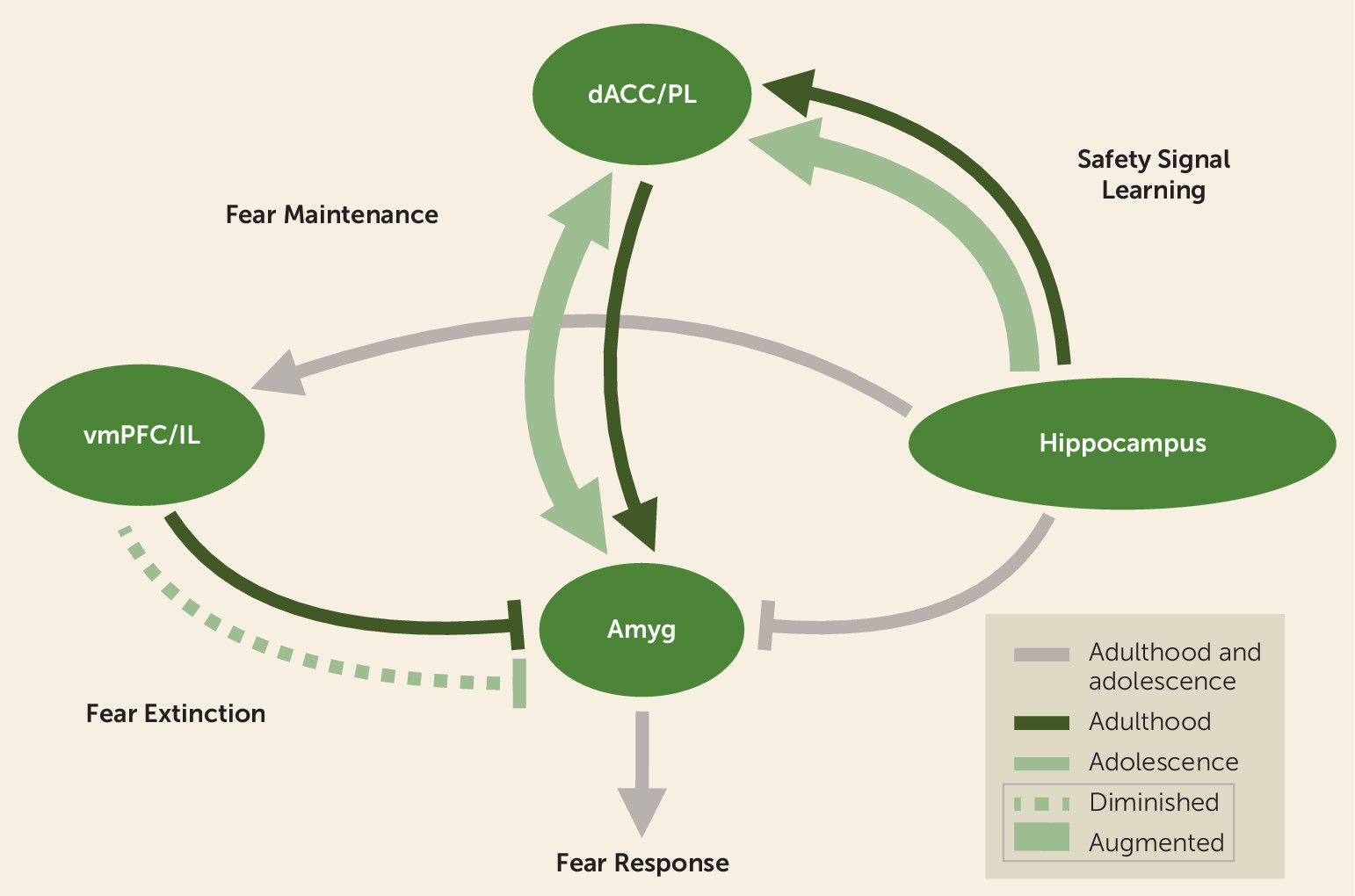
BOX 1. Recommendations for future research on safety signal learning
Clinical Application of Neurobiological Model
Conclusions
Acknowledgments
References
Information & Authors
Information
Published In
History
Keywords
Authors
Competing Interests
Metrics & Citations
Metrics
Citations
Export Citations
If you have the appropriate software installed, you can download article citation data to the citation manager of your choice. Simply select your manager software from the list below and click Download.
For more information or tips please see 'Downloading to a citation manager' in the Help menu.
View Options
View options
PDF/EPUB
View PDF/EPUBGet Access
Login options
Already a subscriber? Access your subscription through your login credentials or your institution for full access to this article.
Personal login Institutional Login Open Athens loginNot a subscriber?
PsychiatryOnline subscription options offer access to the DSM-5-TR® library, books, journals, CME, and patient resources. This all-in-one virtual library provides psychiatrists and mental health professionals with key resources for diagnosis, treatment, research, and professional development.
Need more help? PsychiatryOnline Customer Service may be reached by emailing [email protected] or by calling 800-368-5777 (in the U.S.) or 703-907-7322 (outside the U.S.).