Copy Number Variations in Schizophrenia: Critical Review and New Perspectives on Concepts of Genetics and Disease
Abstract
Objective
Method
Results
Conclusions
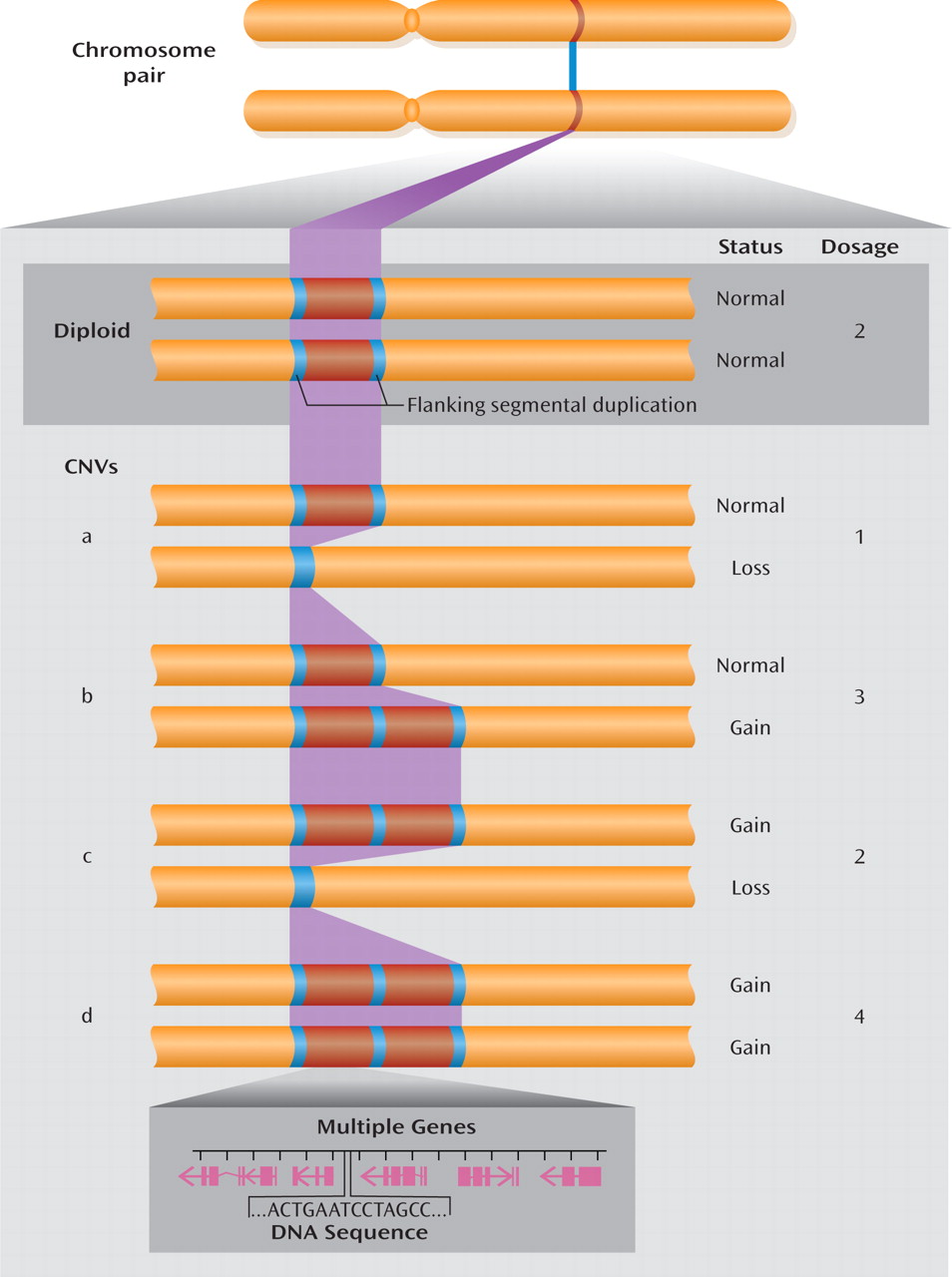
Overview of Copy Number Variations
Detection, Mechanisms, and Expression
Association With Disease
Review Methods
Results
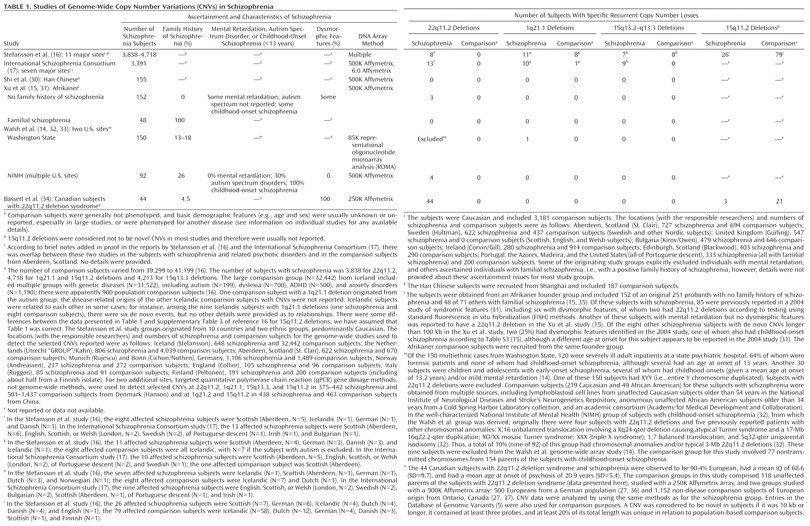
Newly Identified Major CNVs and Lower-Penetrance CNVs Associated With Schizophrenia
Overall Prevalence of CNVs in Schizophrenia
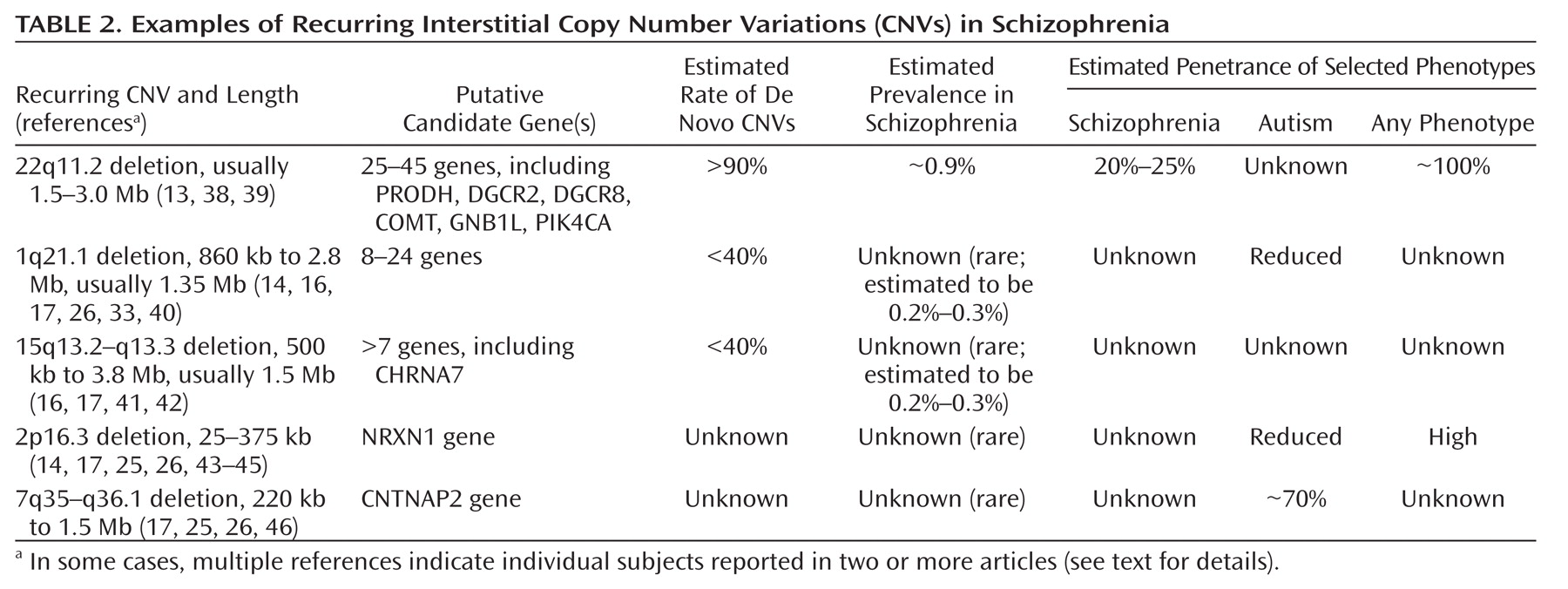
CNVs Implicating Individual Genes
NRXN1
CNTNAP2
Discussion
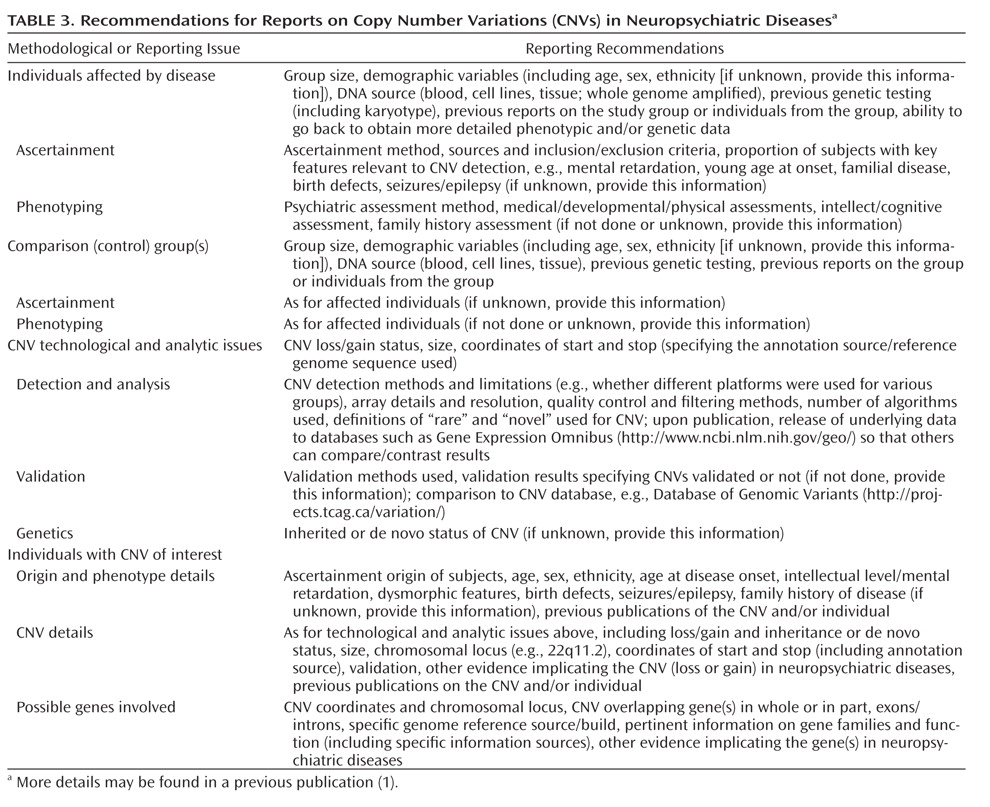
Major Recurrent CNVs Associated With Schizophrenia
22q11.2 deletions
1q21.1 deletions
15q13.3 deletions
Smaller Individual CNVs Implicating Specific Genes
Limitations and Recommendations for Future CNV Studies
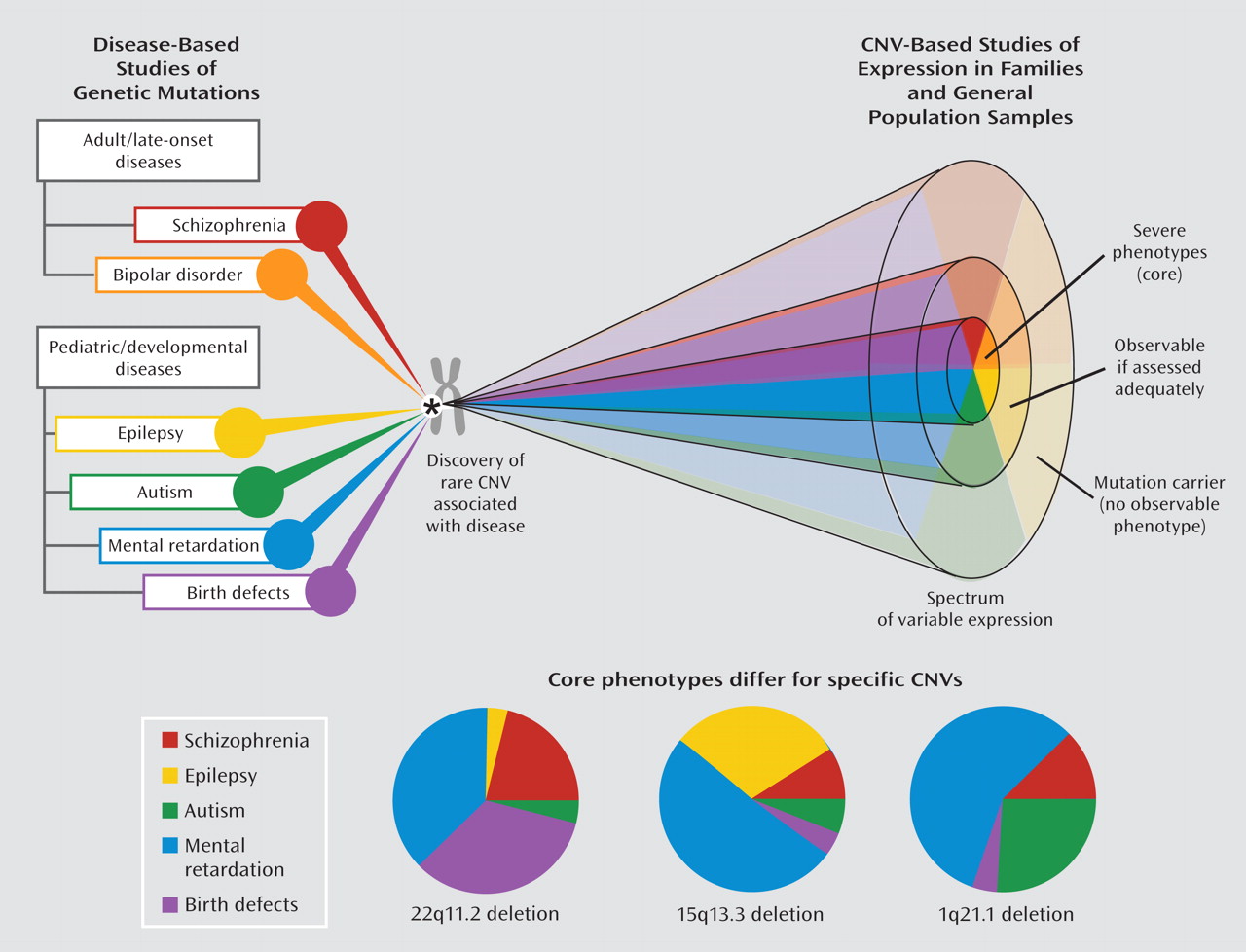
Clinical Relevance of Major CNVs Associated With Genomic Disorders
Neuropsychiatric Perspectives on Phenotype and Implications for Diagnostic Classification
Recurrent De Novo CNVs and a General Mutational Mechanism
Implications for Gene Identification
Conclusions
Acknowledgments
References
Information & Authors
Information
Published In
History
Authors
Competing Interests
Funding Information
Metrics & Citations
Metrics
Citations
Export Citations
If you have the appropriate software installed, you can download article citation data to the citation manager of your choice. Simply select your manager software from the list below and click Download.
For more information or tips please see 'Downloading to a citation manager' in the Help menu.
View Options
View options
PDF/EPUB
View PDF/EPUBLogin options
Already a subscriber? Access your subscription through your login credentials or your institution for full access to this article.
Personal login Institutional Login Open Athens loginNot a subscriber?
PsychiatryOnline subscription options offer access to the DSM-5-TR® library, books, journals, CME, and patient resources. This all-in-one virtual library provides psychiatrists and mental health professionals with key resources for diagnosis, treatment, research, and professional development.
Need more help? PsychiatryOnline Customer Service may be reached by emailing [email protected] or by calling 800-368-5777 (in the U.S.) or 703-907-7322 (outside the U.S.).