Schizophrenia Susceptibility Genes: Emergence of Positional Candidates and Future Directions
Abstract
The genetic component of schizophrenia
Genes identified through the systematic follow-up of linkage signals
Proline dehydrogenase
Dystrobrevin-binding protein 1
Neuregulin 1
G72
Disrupted in schizophrenia 1
C-terminal PDZ ligand of neuronal nitric oxide synthase
ZDHHC8
Trace amine receptor 4
Epsin 4
γ-Aminobutyric acid A receptor subunit gene cluster
Candidate genes located in the vicinity of linkage signals identified through candidate gene approaches
Catechol-O-methyltransferase
Regulator of G-protein signaling 4
Calcineurin γ catalytic subunit
Statistical support and generalization of genetic findings
Future directions of genetic research
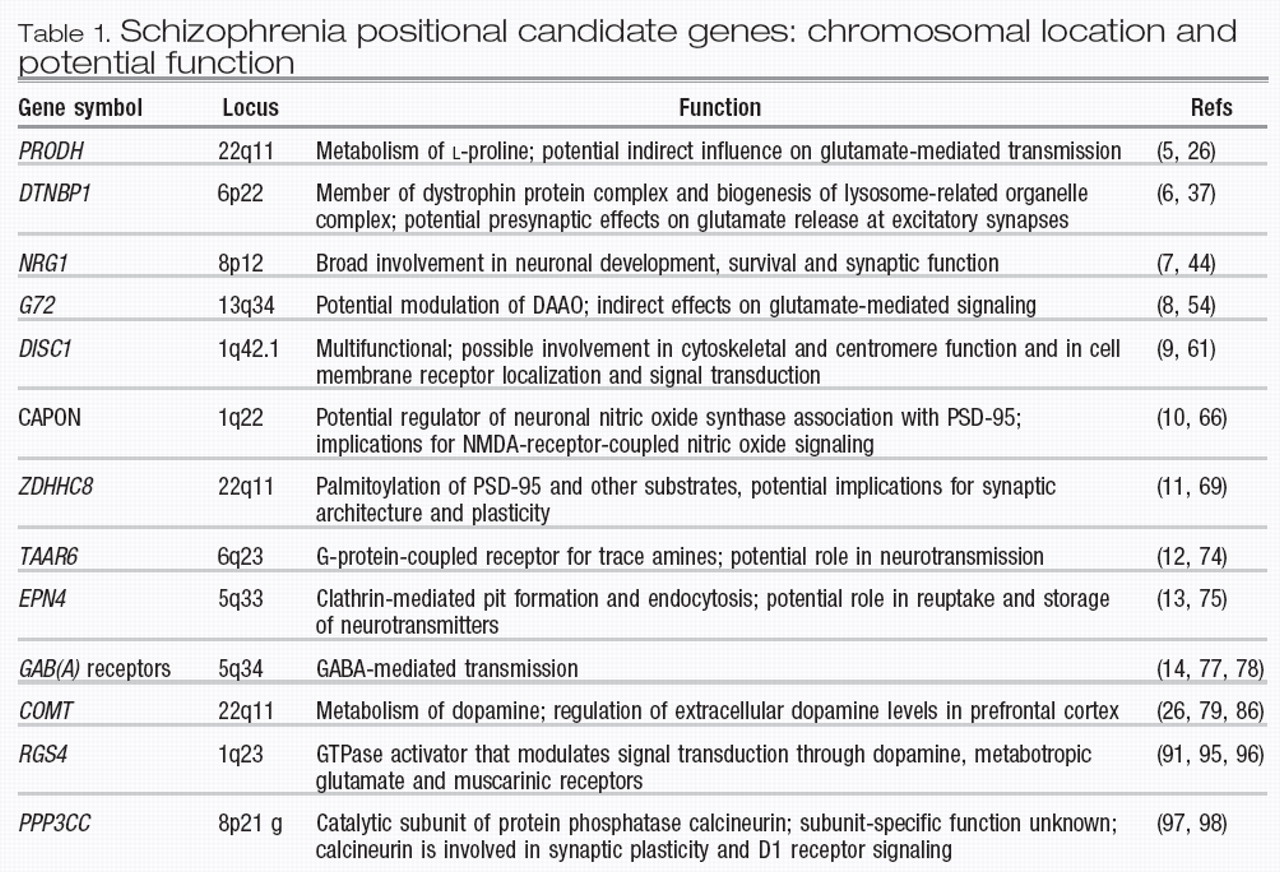
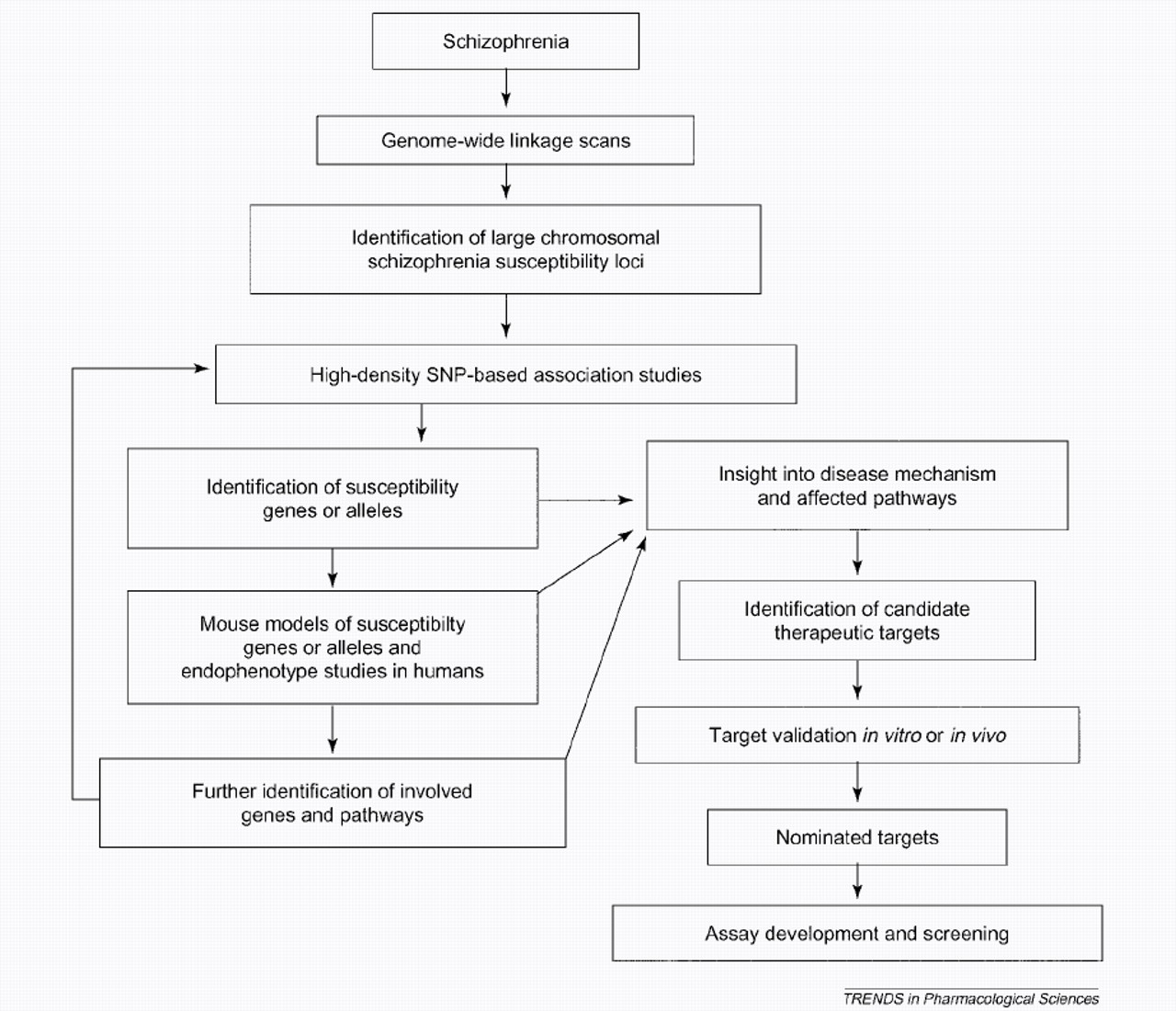
Footnote
References
Information & Authors
Information
Published In
History
Authors
Metrics & Citations
Metrics
Citations
Export Citations
If you have the appropriate software installed, you can download article citation data to the citation manager of your choice. Simply select your manager software from the list below and click Download.
For more information or tips please see 'Downloading to a citation manager' in the Help menu.
View Options
View options
PDF/EPUB
View PDF/EPUBGet Access
Login options
Already a subscriber? Access your subscription through your login credentials or your institution for full access to this article.
Personal login Institutional Login Open Athens loginNot a subscriber?
PsychiatryOnline subscription options offer access to the DSM-5-TR® library, books, journals, CME, and patient resources. This all-in-one virtual library provides psychiatrists and mental health professionals with key resources for diagnosis, treatment, research, and professional development.
Need more help? PsychiatryOnline Customer Service may be reached by emailing [email protected] or by calling 800-368-5777 (in the U.S.) or 703-907-7322 (outside the U.S.).