Discoveries on the Genetics of ADHD in the 21st Century: New Findings and Their Implications
Abstract
The Neurodevelopmental Nature of ADHD
Familial Overlap With Autism and Intellectual Disability
Gene Discoveries and Their Implications for the Conceptualization of ADHD
Rare Genetic Mutations of Strong Effect Sizes That Influence ADHD and Other Neurodevelopmental Disorders
Established syndromes.
Copy number variants.
Sequence variants.
Common Gene Variants: Small Effect Sizes That Have Shared Effects on ADHD and Other Neurodevelopmental Disorders and Traits
ADHD GWAS discoveries to date.
Sex differences.
ADHD overlap with autism.
Genetic Overlap Between ADHD and Other Neuropsychiatric Disorders
Conceptualizing ADHD as a Trait and Category
Twin and Epidemiological Studies
Common Gene Variants
Development and Adult Life
ADHD Trajectories
Adult ADHD
Future Directions
Further Gene Discovery, Biology, and Environment
Implications of Genetic Overlap
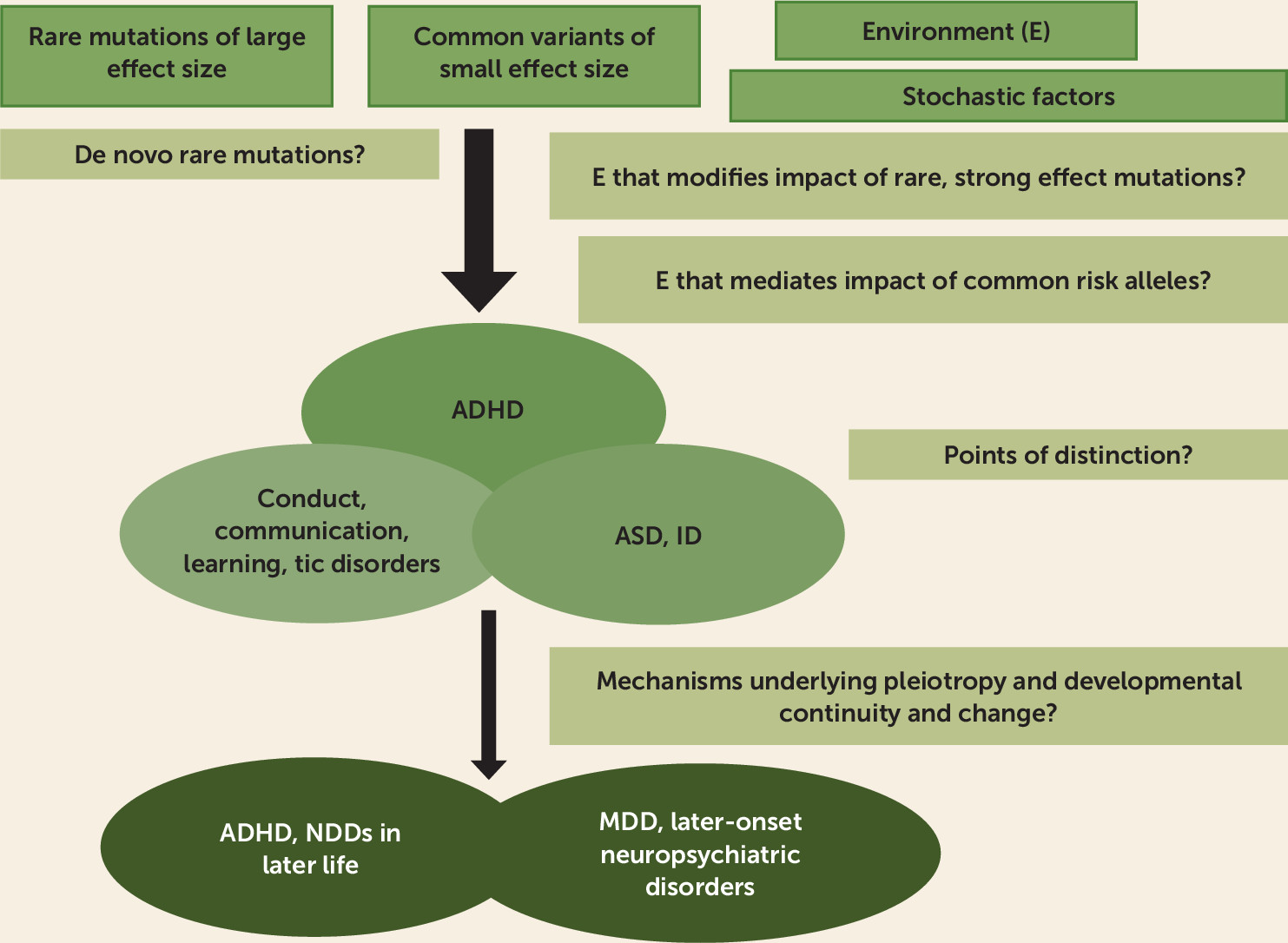
The Developmental Continuum of ADHD
Genetic Testing
Acknowledgments
References
Information & Authors
Information
Published In
History
Keywords
Authors
Competing Interests
Funding Information
Metrics & Citations
Metrics
Citations
Export Citations
If you have the appropriate software installed, you can download article citation data to the citation manager of your choice. Simply select your manager software from the list below and click Download.
For more information or tips please see 'Downloading to a citation manager' in the Help menu.
View Options
View options
PDF/EPUB
View PDF/EPUBLogin options
Already a subscriber? Access your subscription through your login credentials or your institution for full access to this article.
Personal login Institutional Login Open Athens loginNot a subscriber?
PsychiatryOnline subscription options offer access to the DSM-5-TR® library, books, journals, CME, and patient resources. This all-in-one virtual library provides psychiatrists and mental health professionals with key resources for diagnosis, treatment, research, and professional development.
Need more help? PsychiatryOnline Customer Service may be reached by emailing [email protected] or by calling 800-368-5777 (in the U.S.) or 703-907-7322 (outside the U.S.).