Association of the Alzheimer's Gene SORL1 With Hippocampal Volume in Young, Healthy Adults
Abstract
Objective:
Method:
Results:
Conclusions:
Method
Sample
Age (Years) | |||||
---|---|---|---|---|---|
Cohort | N | Mean | SD | Gender (Male/Female) | Single Nucleotide Polymorphisms Analyzed |
Discovery (scanned with 1.5-T) | 446 | 22.9 | 3.54 | 258/188 | 117 |
Replication (scanned with 3.0-T) | 490 | 22.6 | 3.14 | 291/199 | 115 |
Combined | 936 | 22.8 | 3.34 | 549/387 | 117 |
Apolipoprotein E subsample | 735 | 22.8 | 3.28 | 299/436 | 117 |
Genotyping
Neuroimaging Procedures
Brain Segmentation
Analysis
Results
Discovery Cohort (N=446) | Replication Cohort (N=490) | Combined Cohort (N=936) | ||||||
---|---|---|---|---|---|---|---|---|
SNP | Positionb | Unadjusted (p) | Empirical (p) | Unadjusted (p) | Empirical (p) | Unadjusted (p) | Empirical (p) | Type |
rs17125342 | 120813518 | 0.55 | 1.0 | 0.03 | 0.73 | 0.04 | 0.88 | upstream |
rs661057c | 120834164 | 0.94 | 1.0 | 0.01 | 0.39 | 0.05 | 0.89 | intron 2 |
rs1784934 | 120843203 | 0.59 | 1.0 | 0.02 | 0.66 | 0.04 | 0.82 | intron 2 |
rs3781826 | 120848256 | 0.0001 | 0.009 | 0.71 | 1.0 | 0.03 | 0.81 | intron 3 |
rs676759 | 120864475 | 0.21 | 1.0 | 0.001 | 0.07 | 0.001 | 0.06 | intron 5 |
rs560573 | 120866094 | 0.16 | 1.0 | d | d | 0.0004 | 0.02 | intron 6 |
rs593769 | 120869213 | 0.16 | 1.0 | 0.0011 | 0.06 | 0.0006 | 0.03 | intron 6 |
rs12364988 | 120872836 | 0.18 | 1.0 | 0.0024 | 0.13 | 0.0013 | 0.07 | exon 7 |
rs668387c | 120873131 | 0.14 | 1.0 | 0.0003 | 0.02 | 0.0002 | 0.01 | intron 7 |
rs923892 | 120873373 | 0.17 | 1.0 | 0.0003 | 0.02 | 0.0002 | 0.01 | intron 7 |
rs666004 | 120903788 | 0.30 | 1.0 | 0.02 | 0.58 | 0.01 | 0.44 | intron 12 |
rs7933552 | 120922038 | 0.98 | 1.0 | 0.00 | 0.19 | 0.03 | 0.75 | intron 15 |
rs640479 | 120937693 | 0.09 | 1.0 | 0.06 | 0.93 | 0.01 | 0.53 | intron 22 |
rs924746 | 120948247 | 0.02 | 0.70 | 0.09 | 1.0 | 0.01 | 0.32 | intron 24 |
rs3781834 | 120951150 | 0.66 | 1.0 | 0.00 | 0.15 | 0.01 | 0.43 | intron 25 |
rs4420280 | 120951269 | 0.04 | 0.83 | 0.12 | 1.0 | 0.01 | 0.47 | intron 25 |
rs3781836 | 120953548 | 0.89 | 1.0 | 0.00 | 0.20 | 0.05 | 0.89 | intron 26 |
rs1699103 | 120957136 | 0.08 | 0.97 | 0.16 | 1.0 | 0.03 | 0.77 | intron 26 |
rs7116734 | 120957150 | 0.02 | 0.61 | 0.11 | 1.0 | 0.01 | 0.33 | intron 26 |
rs1620003 | 120978203 | 0.07 | 0.96 | 0.15 | 1.0 | 0.03 | 0.71 | intron 33 |
rs1629493 | 120982306 | 0.05 | 0.89 | 0.33 | 1.0 | 0.04 | 0.87 | intron 37 |
rs1784931 | 120988148 | 0.06 | 0.93 | 0.19 | 1.0 | 0.03 | 0.72 | intron 40 |
rs540860 | 121036098 | 0.28 | 1.0 | 0.03 | 0.78 | 0.02 | 0.67 | downstream |
rs663175 | 121039037 | 0.44 | 1.0 | 0.02 | 0.62 | 0.03 | 0.72 | downstream |
rs647905 | 121040148 | 0.40 | 1 | 0.02 | 0.68 | 0.03 | 0.72 | downstream |
rs568599 | 121041221 | 0.33 | 1 | 0.02 | 0.52 | 0.01 | 0.53 | downstream |
rs11218381 | 121067926 | 0.01 | 0.31 | 0.09 | 1 | 0.003 | 0.17 | downstream |
rs10502260 | 121073107 | 0.01 | 0.53 | 0.52 | 1 | 0.03 | 0.80 | downstream |
rs2887763 | 121073389 | 0.02 | 0.58 | 0.04 | 0.85 | 0.003 | 0.17 | downstream |
rs10892771 | 121073472 | 0.04 | 0.87 | 0.02 | 0.53 | 0.003 | 0.15 | downstream |
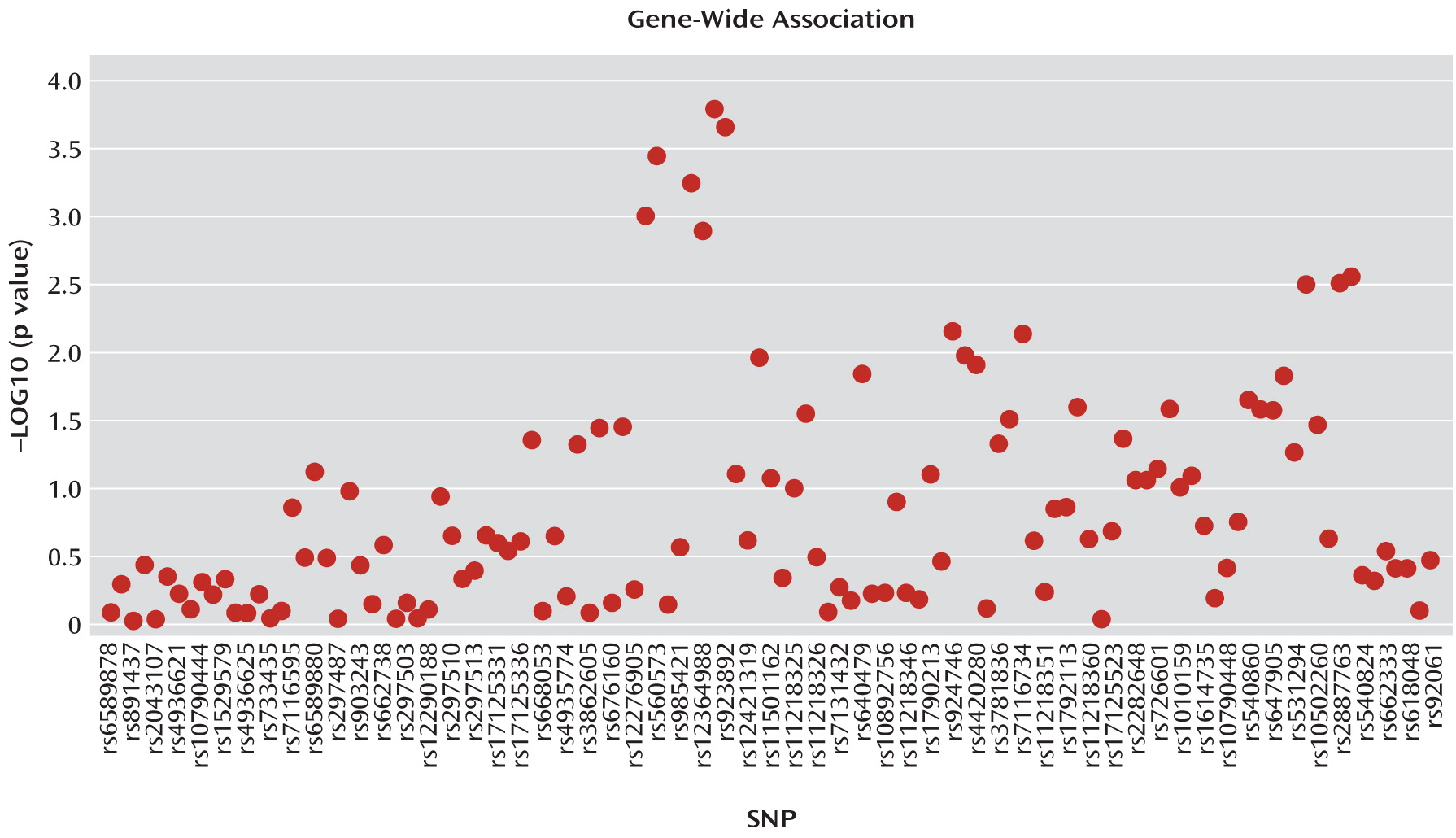
Discussion
Acknowledgments
Footnotes
Supplementary Material
- View/Download
- 164.18 KB
- View/Download
- 54.06 KB
- View/Download
- 9.36 KB
- View/Download
- 148.20 KB
References
Information & Authors
Information
Published In
History
Authors
Author Contributions
Funding Information
Metrics & Citations
Metrics
Citations
Export Citations
If you have the appropriate software installed, you can download article citation data to the citation manager of your choice. Simply select your manager software from the list below and click Download.
For more information or tips please see 'Downloading to a citation manager' in the Help menu.
View Options
View options
PDF/EPUB
View PDF/EPUBGet Access
Login options
Already a subscriber? Access your subscription through your login credentials or your institution for full access to this article.
Personal login Institutional Login Open Athens loginNot a subscriber?
PsychiatryOnline subscription options offer access to the DSM-5-TR® library, books, journals, CME, and patient resources. This all-in-one virtual library provides psychiatrists and mental health professionals with key resources for diagnosis, treatment, research, and professional development.
Need more help? PsychiatryOnline Customer Service may be reached by emailing [email protected] or by calling 800-368-5777 (in the U.S.) or 703-907-7322 (outside the U.S.).