Genome-Wide Association Study of Multiplex Schizophrenia Pedigrees
Abstract
Objective
Method
Results
Conclusions
Method
Subjects
Affected and Unaffected Subjects and Ancestry | ||||||||||||||||
---|---|---|---|---|---|---|---|---|---|---|---|---|---|---|---|---|
Genotyped Affected | Genotyped Unaffected | Families | ||||||||||||||
European | Other | European | Other | Other | ||||||||||||
Site | N | Male | N | Male | N | Male | N | Male | Total | European | Mediterranean | Admix1 | Admix2 | Admix3 | Malabar | Total |
Australia/United States | 128 | 0.63 | 2 | 0.50 | 107 | 0.49 | 1 | 0.00 | 238 | 57 | 1 | 0 | 0 | 0 | 0 | 58 |
Cardiff | 43 | 0.70 | 2 | 0.50 | 30 | 0.33 | 2 | 0.50 | 77 | 21 | 0 | 0 | 0 | 0 | 0 | 21 |
Johns Hopkins | 282 | 0.64 | 6 | 0.67 | 261 | 0.44 | 3 | 0.33 | 552 | 122 | 1 | 0 | 0 | 0 | 0 | 123 |
Illinois/NorthShore | 118 | 0.74 | 2 | 1.00 | 92 | 0.48 | 1 | 0.00 | 213 | 53 | 1 | 0 | 0 | 0 | 0 | 54 |
Paris | 53 | 0.57 | 99 | 0.62 | 37 | 0.54 | 83 | 0.36 | 272 | 21 | 0 | 11 | 11 | 5 | 8 | 56 |
VCU/Ireland | 399 | 0.67 | 0 | — | 286 | 0.43 | 0 | — | 685 | 216 | 0 | 0 | 0 | 0 | 0 | 216 |
Western Australia/Germany | 195 | 0.52 | 28 | 0.57 | 177 | 0.47 | 24 | 0.50 | 424 | 91 | 12 | 0 | 0 | 0 | 0 | 103 |
Total | 1,218 | 0.64 | 139 | 0.61 | 990 | 0.45 | 114 | 0.39 | 2,461 | 581 | 15 | 11 | 11 | 5 | 8 | 631 |
Genotyping, SNP Quality Control, and Genotypic Ancestry
Statistical Analyses of Genetic Association to SNPs
CNV Analysis
Results
Association of Common SNPs
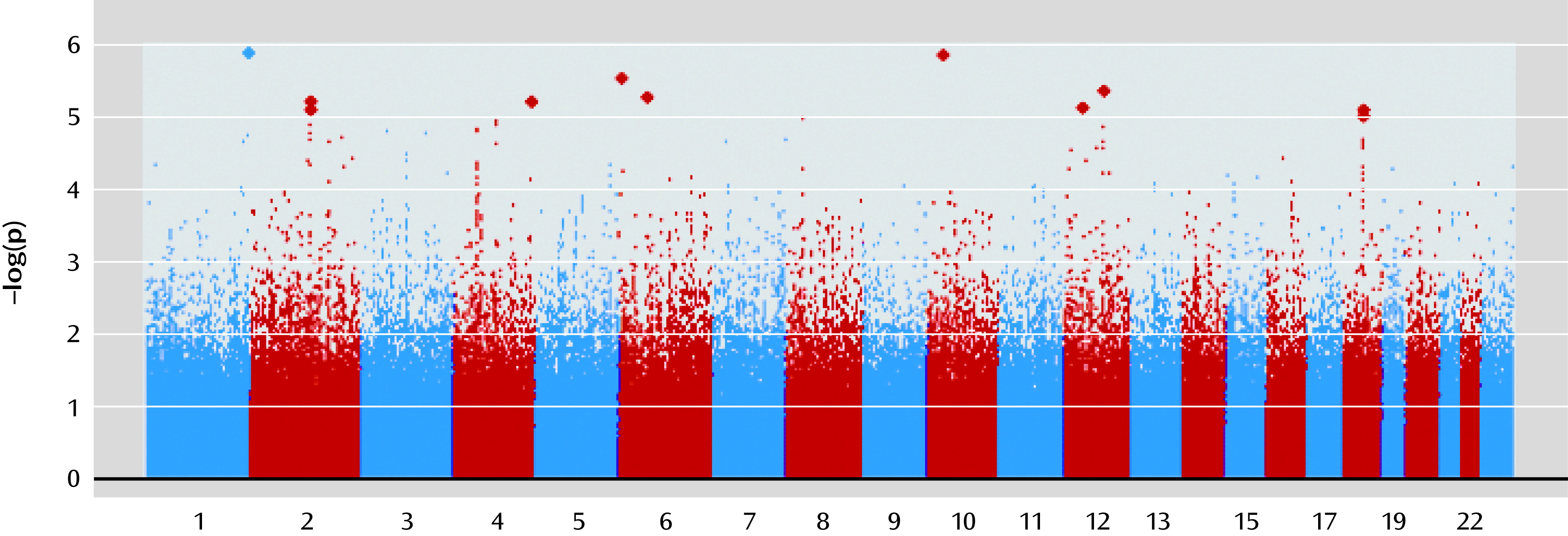
Allele 1 Total Counts | Allele 2 Total Counts | T From Heterozygous Parents | |||||||||||||
---|---|---|---|---|---|---|---|---|---|---|---|---|---|---|---|
SNP | LOC | A1 | Frq | T | NT | A2 | Frq | T | NT | A1 | A2 | Odds Ratio | p | SNPs | Genes (Within 50 kb) |
rs12210050 | chr6:420489 | T | 0.23 | 565 | 459 | C | 0.77 | 1763 | 1869 | 445.1 | 371.7 | 1.20 | 2.9E-06 | 1 | EXOC2,9648 |
rs12426725 | chr12:80367259 | A | 0.15 | 308 | 411 | G | 0.85 | 2030 | 1927 | 254.1 | 347.9 | 0.73 | 4.2E-06 | 4 | PPFIA2 |
rs1170612 | chr2:124699526 | T | 0.22 | 593 | 481 | C | 0.78 | 1745 | 1857 | 475.1 | 339.3 | 1.40 | 5.9E-06 | 7 | CNTNAP5 |
rs16934812 | chr12:29763585 | G | 0.13 | 331 | 248 | T | 0.87 | 2003 | 2086 | 294.0 | 219.6 | 1.34 | 7.2E-06 | 1 | TMTC1 |
rs12511372 | chr4:45811189 | G | 0.50 | 1229 | 1106 | A | 0.50 | 1107 | 1230 | 643.8 | 524.2 | 1.23 | 1.4E-05 | 11 | GABRG1 (and GABRA2 in the SNP cluster) |
rs3197999 | chr3:49696536 | T | 0.30 | 747 | 628 | C | 0.70 | 1587 | 1706 | 539.6 | 437.1 | 1.23 | 1.6E-05 | 1 | BSN,12550; APEH,598; MST1; RNF123,–5457; AMIGO3,33432; GMPPB,37399; IHPK1,40195 |
rs4716801 | chr7:157381124 | G | 0.46 | 1151 | 1022 | A | 0.54 | 1185 | 1314 | 652.2 | 509.0 | 1.28 | 2.1E-05 | 1 | PTPRN2 |
rs7805806 | chr7:20693853 | G | 0.12 | 334 | 250 | A | 0.88 | 2004 | 2088 | 298.9 | 203.8 | 1.47 | 2.2E-05 | 1 | ABCB5 |
rs12239401 | chr1:235261146 | T | 0.44 | 953 | 1080 | C | 0.56 | 1383 | 1256 | 495.2 | 657.5 | 0.75 | 2.2E-05 | 1 | RYR2,–11178 |
rs6433323 | chr2:172581306 | G | 0.38 | 963 | 842 | A | 0.62 | 1375 | 1496 | 623.6 | 479.2 | 1.30 | 2.3E-05 | 2 | HAT1,24460; MAP1D |
rs1037231 | chr3:85845797 | A | 0.42 | 913 | 1038 | G | 0.58 | 1421 | 1296 | 506.2 | 629.0 | 0.80 | 3.3E-05 | 4 | CADM2,–12524 |
rs3892156 | chr16:48877496 | A | 0.25 | 659 | 552 | G | 0.75 | 1669 | 1776 | 509.5 | 371.3 | 1.37 | 3.7E-05 | 1 | ADCY7,–1827; BRD7,32945 |
rs2396465 | chr2:228234344 | G | 0.10 | 267 | 198 | A | 0.90 | 2071 | 2140 | 244.6 | 168.1 | 1.46 | 3.8E-05 | 1 | DKFZp547H025,–28212; SLC19A3,23825 |
rs12565770 | chr1:19427647 | A | 0.12 | 229 | 312 | G | 0.88 | 2109 | 2026 | 197.6 | 281.8 | 0.70 | 4.5E-05 | 1 | UBR4,–18314; KIAA0090; MRTO4,–23014; AFAR3,37415 |
rs1851185 | chr2:212235974 | T | 0.24 | 593 | 490 | C | 0.76 | 1745 | 1848 | 462.8 | 380.3 | 1.22 | 4.8E-05 | 1 | ERBB4 |
rs12321966 | chr12:8592432 | T | 0.09 | 264 | 196 | G | 0.91 | 2072 | 2140 | 243.5 | 153.6 | 1.58 | 5.1E-05 | 1 | CLEC4D,26205; CLEC4E,–7607 |
rs4805453 | chr19:34814743 | C | 0.42 | 1050 | 927 | T | 0.58 | 1286 | 1409 | 635.7 | 503.2 | 1.26 | 5.3E-05 | 1 | POP4,16196; PLEKHF1,–33423 |
rs6901207 | chr6:3798905 | G | 0.44 | 1110 | 995 | A | 0.56 | 1228 | 1343 | 650.3 | 503.8 | 1.29 | 5.6E-05 | 1 | FAM50B,2355 |
rs6443997 | chr3:186016225 | A | 0.06 | 106 | 156 | G | 0.94 | 2232 | 2182 | 98.0 | 159.2 | 0.62 | 6.1E-05 | 1 | VPS8 |
rs795955 | chr12:77160181 | T | 0.40 | 883 | 993 | C | 0.60 | 1455 | 1345 | 503.7 | 621.1 | 0.81 | 6.2E-05 | 1 | NAV3,29260 |
rs10507070 | chr12:94873188 | A | 0.17 | 451 | 359 | G | 0.83 | 1885 | 1977 | 381.6 | 285.1 | 1.34 | 6.3E-05 | 1 | CCDC38,–12629; AMDHD1; HAL,18084; LTA4H,45553 |
rs7179849 | chr15:22589304 | T | 0.18 | 383 | 475 | C | 0.82 | 1955 | 1863 | 305.7 | 389.8 | 0.78 | 6.6E-05 | 1 | SNRPN,–30582 |
rs7180015 | chr15:85305969 | G | 0.09 | 162 | 226 | A | 0.91 | 2176 | 2112 | 144.4 | 225.8 | 0.64 | 7.1E-05 | 1 | AGBL1 |
rs1782 | chr6:90124434 | C | 0.12 | 311 | 240 | T | 0.88 | 2017 | 2088 | 279.7 | 197.7 | 1.41 | 7.7E-05 | 1 | GABRR2,–42748; UBE2J1,–5096; RRAGD,9878 |
rs2362643 | chr16:68503033 | G | 0.32 | 815 | 701 | A | 0.68 | 1523 | 1637 | 578.2 | 436.4 | 1.32 | 7.8E-05 | 1 | WWP2; LOC348174,–39277 |
rs2211871 | chr21:38744520 | G | 0.09 | 242 | 179 | T | 0.91 | 2094 | 2157 | 222.4 | 166.6 | 1.33 | 8.3E-05 | 2 | ERG |
rs4925449 | chr22:47486086 | A | 0.08 | 170 | 237 | G | 0.92 | 2166 | 2099 | 153.4 | 207.6 | 0.74 | 8.8E-05 | 1 | FAM19A5 |
rs175 | chr7:25000316 | C | 0.47 | 1142 | 1031 | A | 0.53 | 1194 | 1305 | 635.4 | 527.1 | 1.21 | 9.2E-05 | 1 | OSBPL3,–14031 |
rs10760120 | chr9:99908721 | G | 0.47 | 1024 | 1143 | A | 0.53 | 1308 | 1189 | 514.3 | 646.8 | 0.80 | 9.3E-05 | 1 | NANS,23543; TRIM14; CORO2A,17575 |
rs10489577 | chr1:231021449 | C | 0.04 | 103 | 61 | T | 0.96 | 2235 | 2277 | 100.0 | 62.3 | 1.60 | 9.5E-05 | 1 | KIAA1383,8734 |
rs921383 | chr11:77388489 | A | 0.47 | 1172 | 1057 | G | 0.53 | 1162 | 1277 | 656.4 | 506.4 | 1.30 | 9.8E-05 | 1 | INTS4,–5124; KCTD14,15919 |
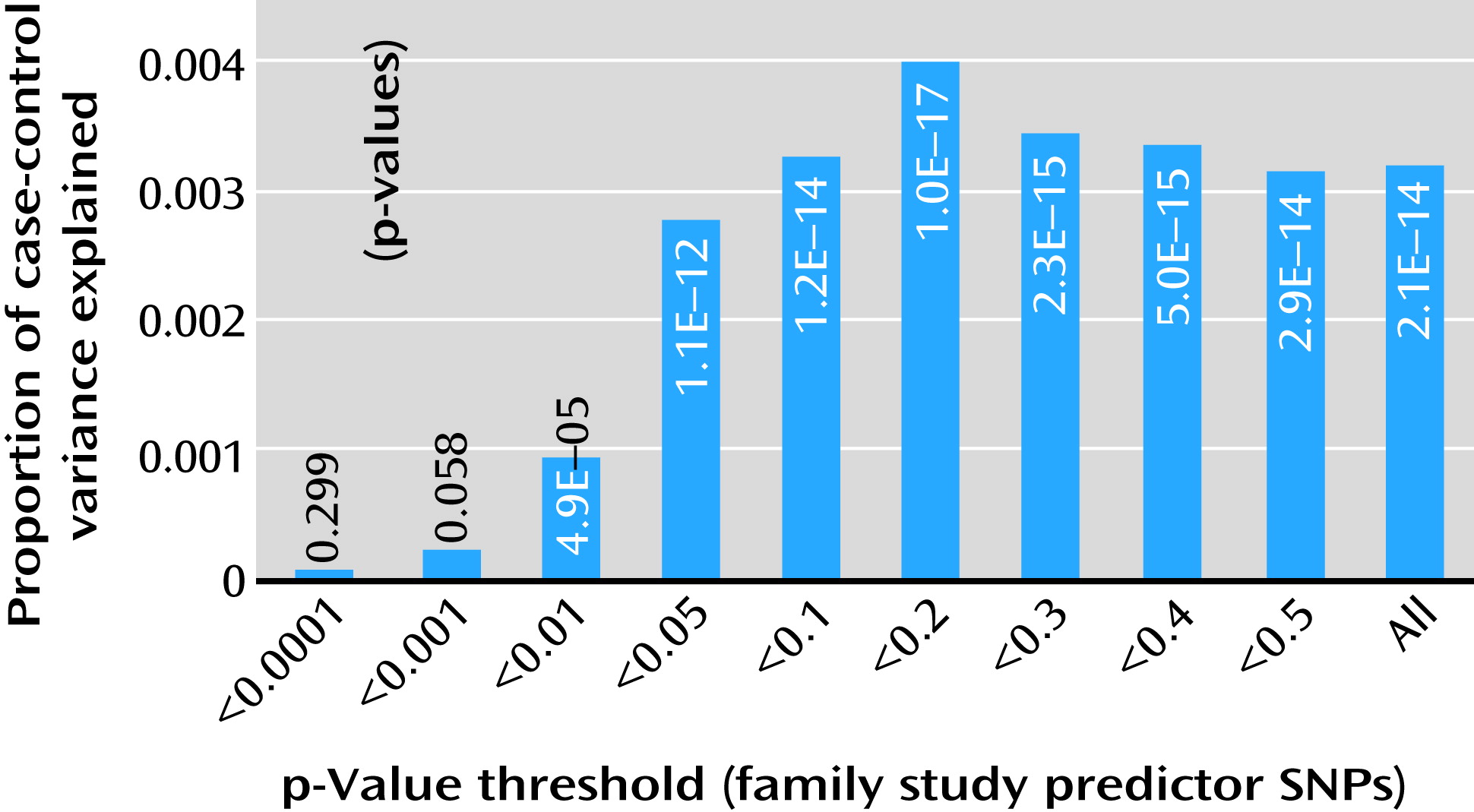
Previously Documented CNV Regions
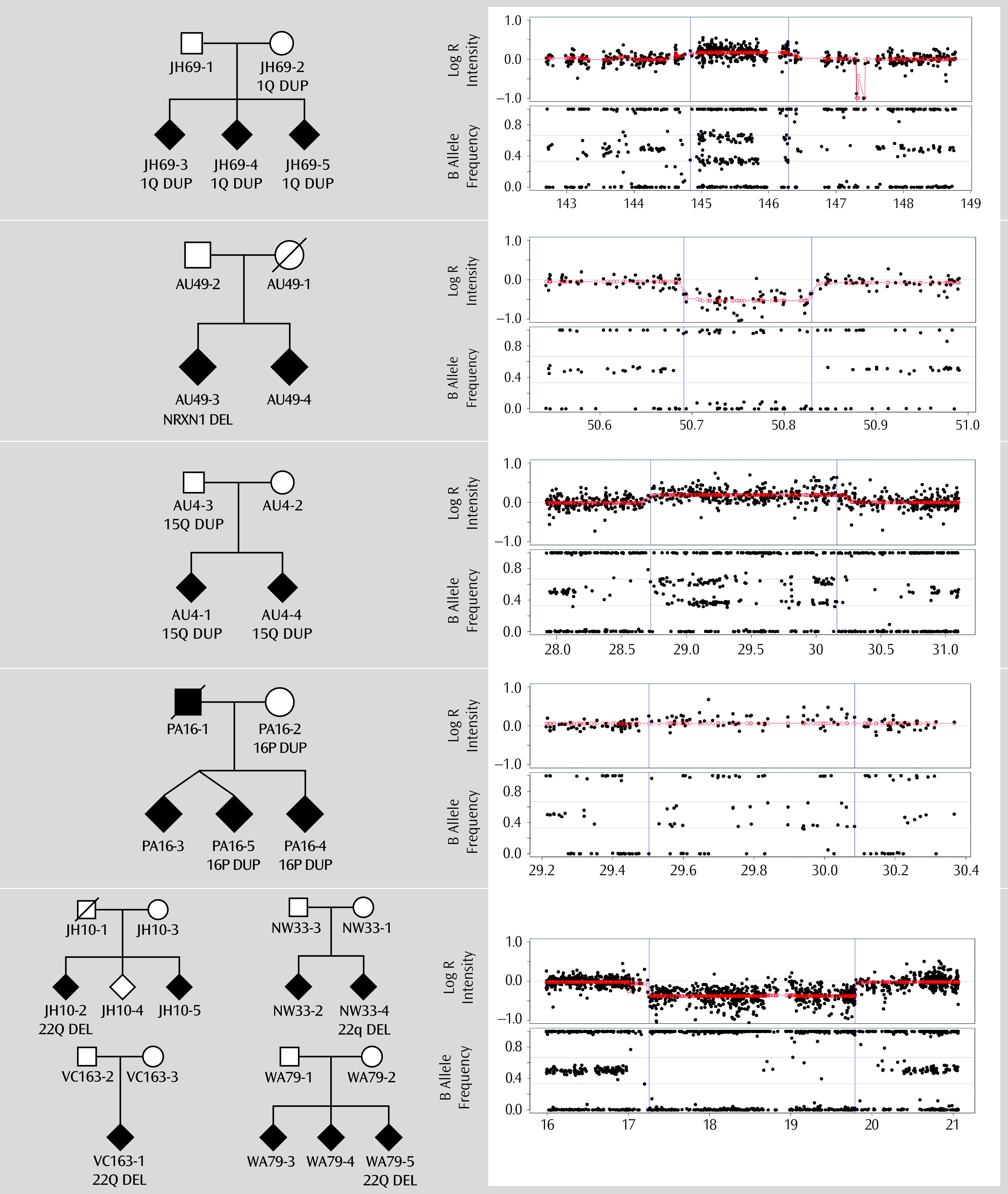
Discussion
Conclusions
Acknowledgments
Footnote
Supplementary Material
- View/Download
- 466.26 KB
References
Information & Authors
Information
Published In
History
Authors
Funding Information
Metrics & Citations
Metrics
Citations
Export Citations
If you have the appropriate software installed, you can download article citation data to the citation manager of your choice. Simply select your manager software from the list below and click Download.
For more information or tips please see 'Downloading to a citation manager' in the Help menu.
View Options
View options
PDF/EPUB
View PDF/EPUBLogin options
Already a subscriber? Access your subscription through your login credentials or your institution for full access to this article.
Personal login Institutional Login Open Athens loginNot a subscriber?
PsychiatryOnline subscription options offer access to the DSM-5-TR® library, books, journals, CME, and patient resources. This all-in-one virtual library provides psychiatrists and mental health professionals with key resources for diagnosis, treatment, research, and professional development.
Need more help? PsychiatryOnline Customer Service may be reached by emailing [email protected] or by calling 800-368-5777 (in the U.S.) or 703-907-7322 (outside the U.S.).