Evidence That Altered Amygdala Activity in Schizophrenia Is Related to Clinical State and Not Genetic Risk
Abstract
Method
Participants
Experimental Paradigm
Data Acquisition
Demographic and Behavioral Data
fMRI
Functional Connectivity Analysis
Statistical Inference
Correlations With Variables Related to the State of Disease
Genotyping
Results
Demographic and Behavioral Data
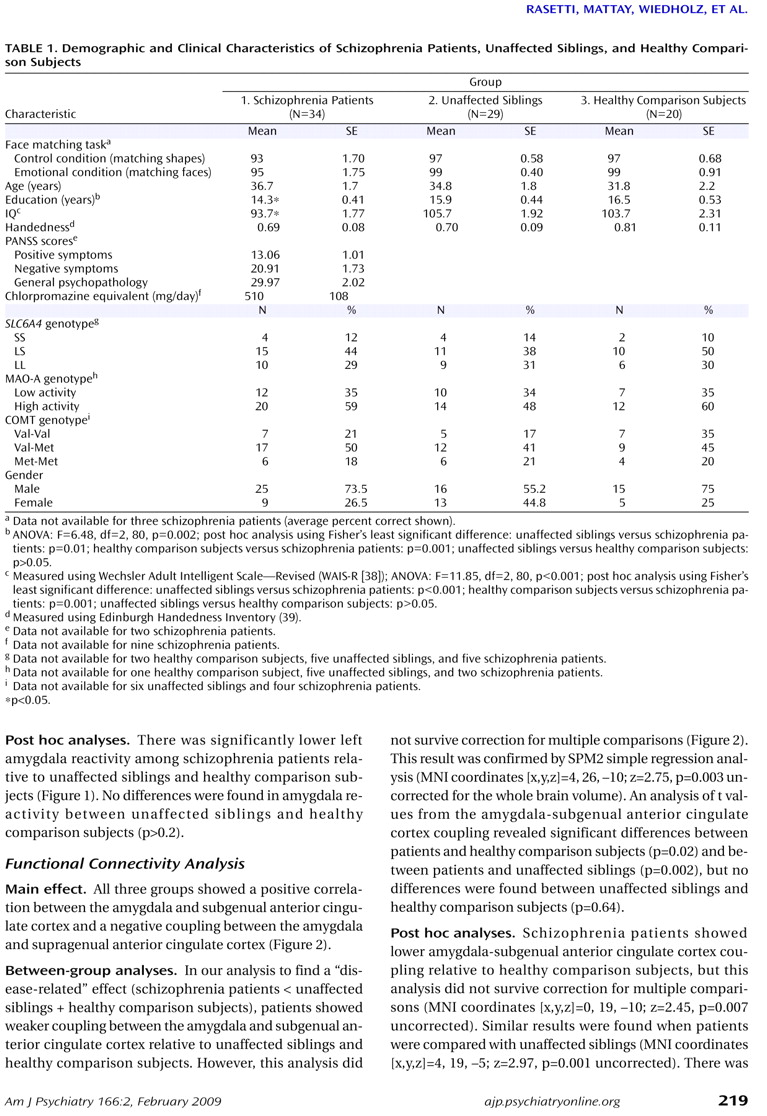
BOLD fMRI
Main effect of the task
Between-group analyses
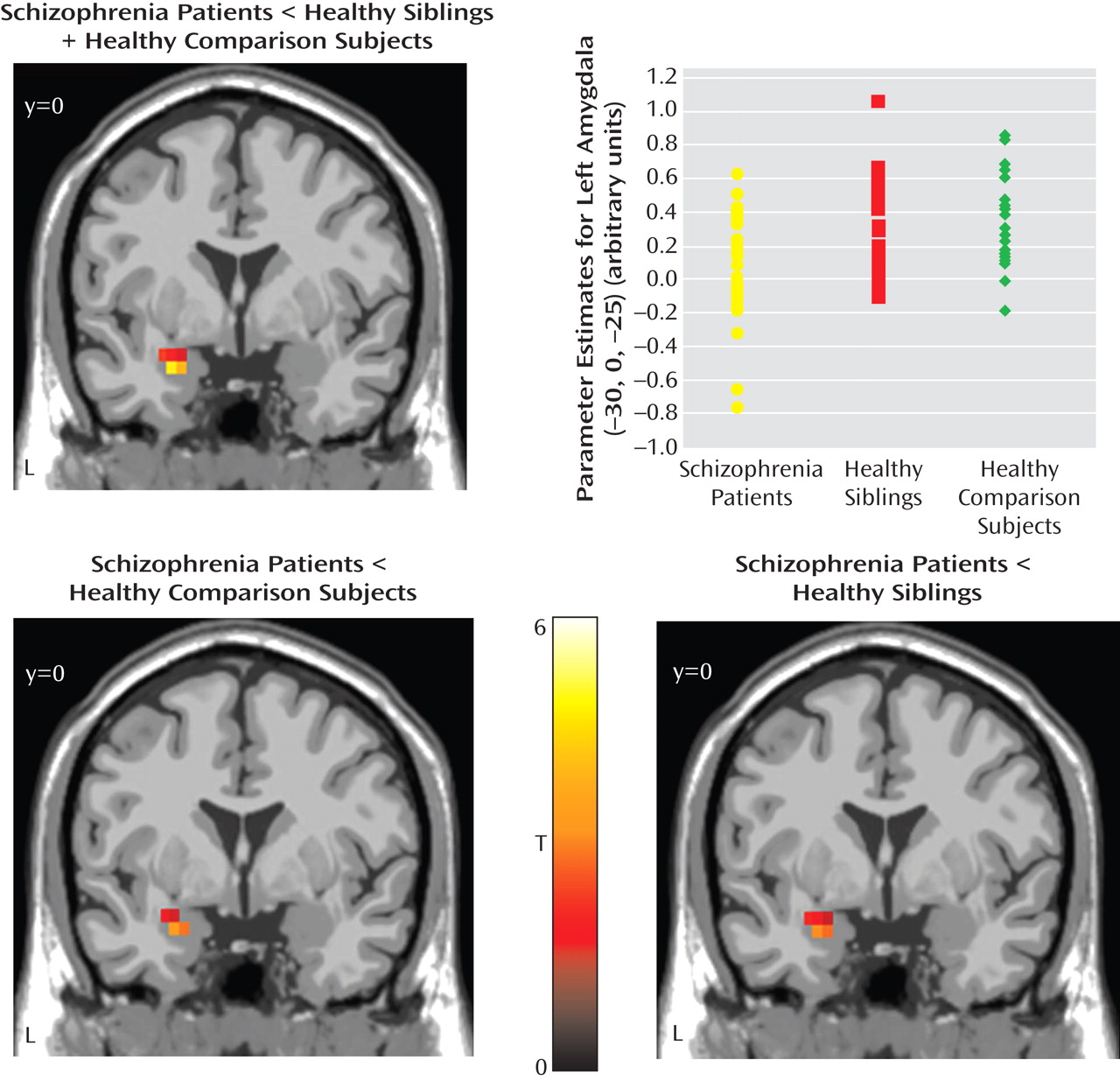
Post hoc analyses
Functional Connectivity Analysis
Main effect
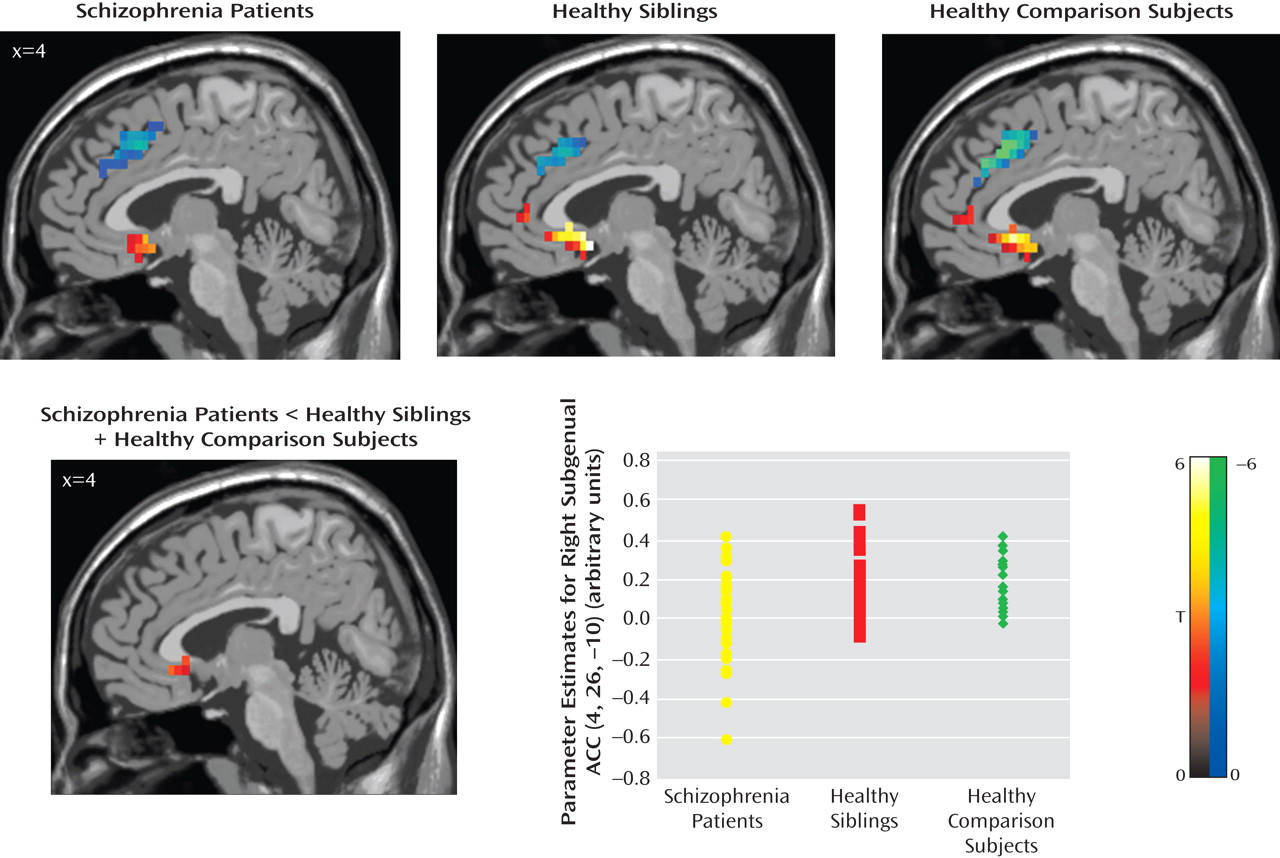
Between-group analyses
Post hoc analyses
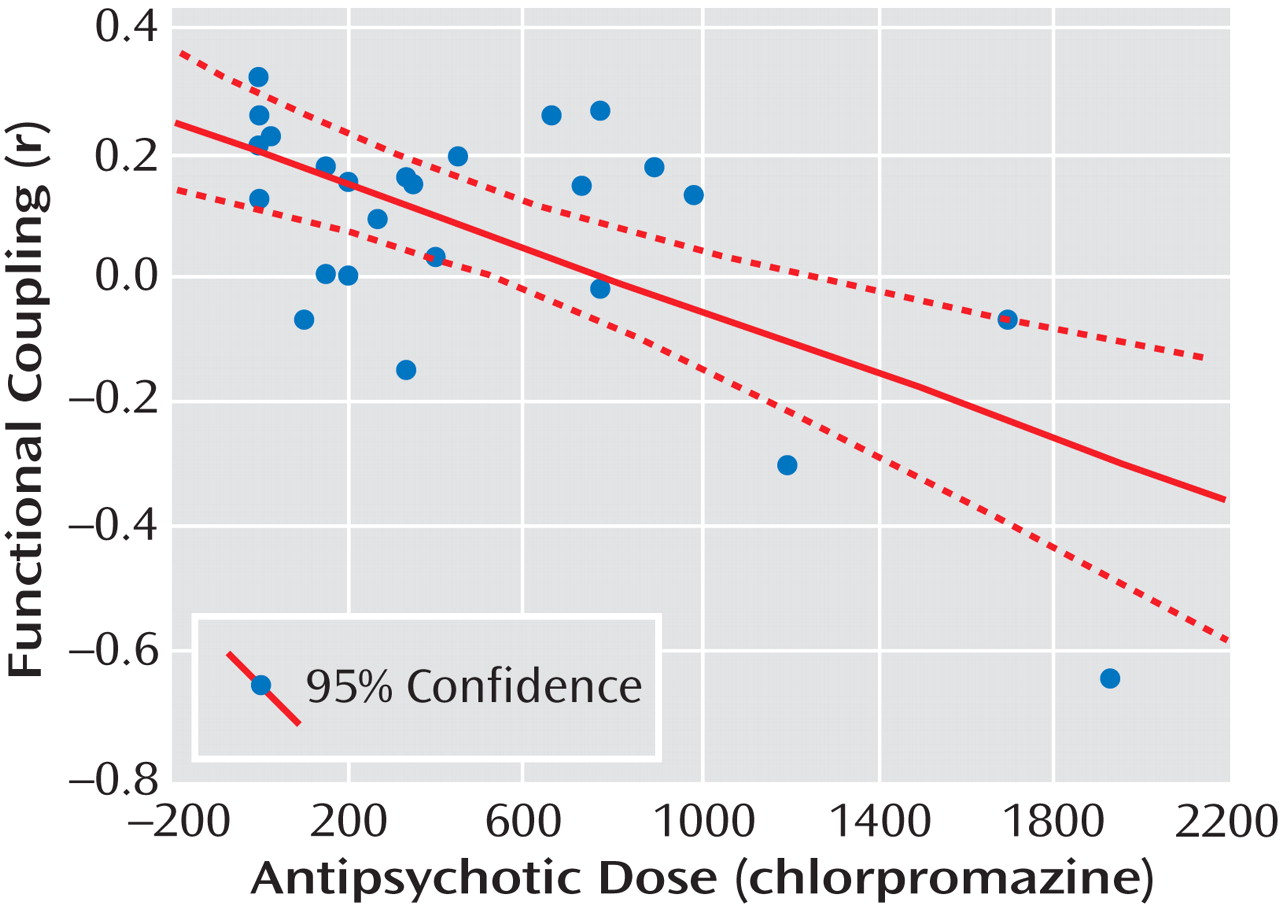
Analysis in a Subsample of Male Subjects
N-Back Working Memory Task
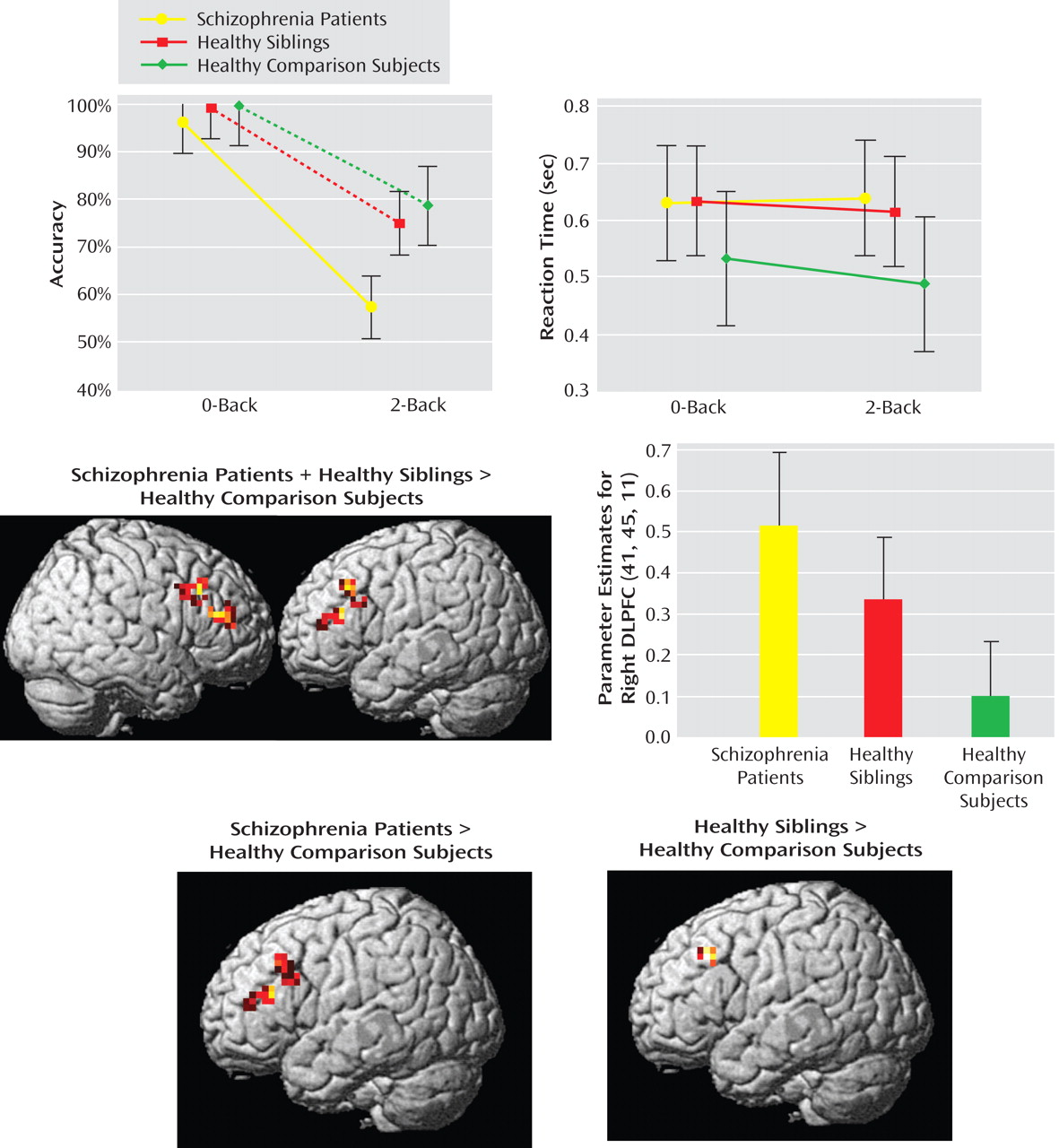
Discussion
Footnotes
References
Information & Authors
Information
Published In
History
Authors
Metrics & Citations
Metrics
Citations
Export Citations
If you have the appropriate software installed, you can download article citation data to the citation manager of your choice. Simply select your manager software from the list below and click Download.
For more information or tips please see 'Downloading to a citation manager' in the Help menu.
View Options
View options
PDF/EPUB
View PDF/EPUBLogin options
Already a subscriber? Access your subscription through your login credentials or your institution for full access to this article.
Personal login Institutional Login Open Athens loginNot a subscriber?
PsychiatryOnline subscription options offer access to the DSM-5-TR® library, books, journals, CME, and patient resources. This all-in-one virtual library provides psychiatrists and mental health professionals with key resources for diagnosis, treatment, research, and professional development.
Need more help? PsychiatryOnline Customer Service may be reached by emailing [email protected] or by calling 800-368-5777 (in the U.S.) or 703-907-7322 (outside the U.S.).