Neural Circuitry of Impaired Emotion Regulation in Substance Use Disorders
Abstract
Four Dimensions Underlying Emotion Regulation
Affect Intensity/Reactivity
Affective Modulation and Cognitive Modulation
Behavioral Control
Neural Circuitry of Emotion Regulation
Selected Regions of Focus
Region of Focus and Region With Functional Connections | Structurally Connected via |
---|---|
Emotion-generating and emotion-processing regions | |
Amygdalab: assigns value to positive and negative emotional cues, is involved in fear conditioning, activates during stress and exposure to negative stimuli, generates fear response (21, 44) | |
Insula | Ventrolateral branch of UF; ventral amygdalofugal pathway |
dmPFC | Anteromedial branch of the UF; external capsule; cingulum |
rACC/vmPFC | UF; cingulum; anterior corona radiata via the internal capsule; ventral amygdalofugal pathway; inferior thalamic peduncle/radiation |
lPFC | Ventrolateral branch of UF; external capsule; cingulum |
Amygdala | AC (interhemispheric connections) |
Insula (SN)c: activates in response to salient stimuli (positive and negative cues), processes interoceptive information (ascending visceral inputs to insula) (42), activates during stress and exposure to negative stimuli, is involved in fear conditioning (21, 44) | |
Amygdala | Ventrolateral branch of UF; ventral amygdalofugal pathway |
dmPFC | Superior fronto-occipital fasciculus |
rACC/vmPFC | Unnamed tracts (structural connectivity demonstrated through seed-based studies) |
lPFC | Extreme capsule; short association fibers (fronto-insular tracts) |
Insula | Midbody of the CC (interhemispheric connections) |
Regulatory regions | |
dmPFCd (includes dACC, preSMA, SMA) (CCN/ECN): activates in response to salient stimuli (positive and negative cues), is involved in performance monitoring/error monitoring, conflict processing, integrating emotional response during goal selection, response conflict, response execution (preSMA/SMA) (24, 41, 42, 44) | |
lPFC | Short association fibers (frontal aslant tract) (intrahemispheric connections) |
dmPFC | Regions of the CC (genu, rostrum, rostral body, anterior midbody, midbody) (interhemispheric connections) |
rACC/vmPFC | Short association fibers (interhemispheric connections) |
lPFCe (includes dlPFC, vlPFC, lOFC) (CCN/ECN): involved in planning, selection of goals, sequencing, holding information online (dlPFC), response inhibition (especially vlPFC/lOFC), conscious/voluntary regulation of amygdala and insula activation (24, 41, 42, 44) | |
dmPFC | Short association fibers (frontal aslant tract) (intrahemispheric connections) |
lPFC | Regions of the CC (genu, rostrum, rostral body, anterior midbody) (interhemispheric connections) |
rACC/vmPFC | Short association fibers (interhemispheric connections) |
rACC/vmPFCf (includes rACC, vmPFC, mOFC, pgACC, sgACC) (DMN): is involved in the subjective valuation of cues (assigns motivational salience and encodes outcome expectancies during emotional decision making, determines motivational priorities), is involved in self-referential introspection (tags information as personally relevant), processes emotional conflict (15, 48), mediates extinction (16), provides automatic/unconscious regulation of amygdala and insula activation (15, 24) | |
dmPFC | Regions of the CC (genu, rostrum, rostral body) (interhemispheric connections) |
rACC | Frontal forceps (interhemispheric connections) |
lPFC | Short association fibers (frontal orbitopolar tract) (intrahemispheric connections) |
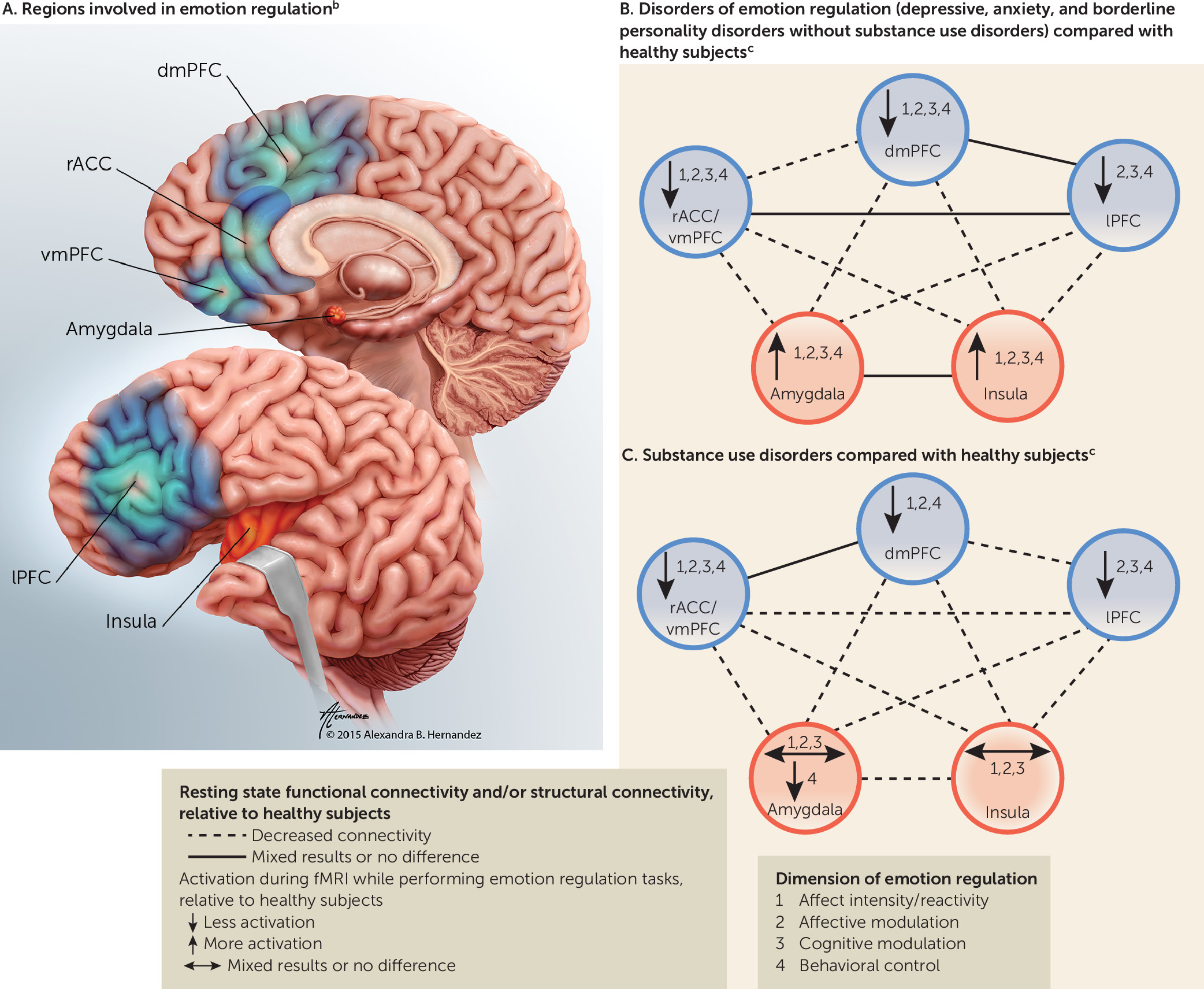
Neural Circuitry of Emotion Regulation During fMRI Tasks
Neural Circuitry in Disorders of Negative Affect
fMRI Tasks of Emotion Regulation
Affect intensity/reactivity.
Affective modulation.
Cognitive modulation.
Behavioral control.
Resting State Functional Connectivity and Structural Connectivity
Neural Circuitry of Emotion Regulation in Substance Use Disorders
Study | Task | Stress Versus Baseline Contrast | Stress Versus Neutral Contrast | Overall Effect: Neutral Plus Stressc | Neutral Trials Only | Regions |
---|---|---|---|---|---|---|
Affect intensity/reactivity tasks | ||||||
Gilman et al. 2008 (17) | Negative or neutral pictures paired with alcohol or neutral beverages | AUD > controls | R amygdala | |||
O’Daly et al. 2012d (73) | Fearful faces | AUD < controls | R amygdala | |||
AUD < controls | B insula (AUD with history of multiple detoxifications < AUD with history of single detoxification < controls); B middle/lOFC (AUD with history of multiple detoxifications < AUD with history of single detoxification and controls) | |||||
Salloum et al. 2007 (72) | Negative emotional faces | AUD < controls | Fear: L vmPFC/middle OFC, L rACC, L insula, R sgACC; disgust: L dlPFC, L dACC, L rACC, R amygdala, R insula | |||
AUD > controls | Disgust: L dlPFC; anger: L insula | |||||
Affective modulation tasks | ||||||
Potenza et al. 2012 (75) | Personalized script | Women only: cocaine use disorder > controls | B insula, dACC, L dlPFC, B amygdala, B posterolateral OFC (BA 47) | |||
Women only: cocaine use disorder > controls | L vlPFC/B posterolateral OFC (BA 47), vmPFC, B dlPFC, rACC | |||||
Men only: cocaine use disorder > controls | L insula, dACC | |||||
Men only: cocaine use disorder > controls | sgACC, rACC, B vmPFC, dACC, B insula, B amygdala, B vlPFC (BA 47), B dlPFC (lateral BA 10) | |||||
Seo et al. 2013 (26) | Personalized script | AUD < controls | L insula, dmPFC (BA 8, 9), L lOFC, B dlPFC (BA 6, 8), vmPFC, sgACC | |||
AUD > controls | vmPFC, sgACC | |||||
AUD < controls | L lOFC, L insula, L dlPFC (BA 6, 8) | |||||
Worse-outcome AUD < better-outcome AUD | rACC, vmPFC, R vlPFC | |||||
Worse-outcome AUD > better-outcome AUD | sgACC, vmPFC, rACC | |||||
Sinha et al. 2005 (25) | Personalized script | Cocaine use disorder < controls | dACC | |||
Sinha et al. 2007 (118) | Personalized script | Worse-outcome cocaine use disorder > better-outcome cocaine use disorder | dmPFC/frontal pole (BA 9, 10) | |||
Wang et al. 2010 (28) | Short negative stimuli (IAPS) in block design | Opioid use disorder < controls | R amygdala | |||
Xu et al. 2013 (119) | Personalized script | Cocaine use disorder with higher-relapse-risk genotype (CG) > cocaine use disorder with lower-relapse-risk genotype (CC) | R amygdala | |||
Yang et al. 2013 (74) | Conditioned paradigm | AUD < controls (AUD deactivated to high threat, controls did not) | rACC | |||
AUD < controls (AUD deactivated more than controls to high threat) | rACC | |||||
AUD > controls (controls deactivated more than AUD to low threat) | rACC | |||||
Cognitive modulation tasks | ||||||
Albein-Urios et al. 2014 (76) | Reappraisal | Cocaine use disorder < controls | L dlPFC, L insula | |||
Behavioral control tasks | ||||||
Smoski et al. 2011 (38) | Emotional oddball task | Opioid use disorder < controls | B amygdala, dACC, R dlPFC, L sgACC | |||
Opioid use disorder < controls | B amygdala, dACC | |||||
Opioid use disorder > controls | dACC | |||||
Opioid use disorder > controls | L IFG/vlPFC (BA 45) |
Study | Seeds | Connectivity | Regions |
---|---|---|---|
Camchong et al. 2013 (80) | sgACC | Worse-outcome AUD < better-outcome AUD | sgACC–L dlPFC, sgACC–B insula |
Gu et al. 2010 (77) | B amygdala, B rACC | Cocaine use disorder < controls | B amygdala–rACC, B rACC–R insula, B rACC–B amygdala |
McHugh et al. 2014 (70) | L and R BL amygdala, L and R CM amygdala | Worse-outcome cocaine use disorder < better-outcome cocaine use disorder and controls | L CM amygdala–vmPFC/rACC |
Müller-Oehering et al. 2014 (81) | dACC, B dlPFC | AUD > controls | dACC–B vmPFC |
AUD < controls | B dlPFC–R dACC/insula | ||
O’Daly et al. 2012 (73) | R and L insula, R and L amygdala | AUD < controls | L insula–L rACC (AUD with history of multiple detoxifications versus controls), L insula–L vmPFC (AUD with history of multiple detoxifications versus controls), L insula–R vlPFC (AUD with history of multiple detoxifications versus controls) |
AUD > controls | L insula–R vlPFC (AUD with history of single detoxification versus controls), L insula–L vmPFC (AUD with history of single detoxification versus controls) | ||
Positive correlation with number of past detoxifications (more detoxifications > fewer detoxifications) | L amygdala–L dlPFC | ||
Negative correlation with number of past detoxifications (more detoxifications < fewer detoxifications) | L insula–L vlPFC | ||
Pujol et al. 2014 (79) | R and L insula, but authors report only R because L was similar | Cannabis use disorder < controls | R insula–dACC, R insula–rACC/vmPFC (more anticorrelated in cannabis use disorder than in controls) |
Sutherland et al. 2013 (71) | R and L insulac | Nicotine use disorder with greater alexithymia and craving in withdrawal < nicotine use disorder with lower alexithymia and craving in withdrawal | R insula–sgACC/rACC |
Upadhyay et al. 2010 (78) | B insulad, B BL amygdala, B CM amygdala | Opioid use disorder < controls | B insula–B lOFC, B insula–vmPFC, B insula–B BL amygdala, B insula–dACC, B CM amygdala–rACC, B BL amygdala–B lOFC, B BL amygdala–dmPFC |
Study | Tracts/Volumes of Interest (and Diffusion Tensor Imaging Measures) | Structural Connectivity | Substance Use Disorder Group | Control Group | Exclusion Criteria |
---|---|---|---|---|---|
Alhassoon et al. 2012 (88) | Body of CC, genu of CC (fractional anisotropy, radial diffusivity) | AUD < controls | 15 abstinent AUD (15 male; mean age=51.4, SD=6); minimum 2 weeks abstinent; 11 smokers; 0 with other SUD | 15 controls (15 male; mean age=51.8, SD=7.4); 3 smokers; 0 with SUD | Any axis I disorder except major depressive disorder, other SUD except nicotine or cannabis, not having “urine and blood assured sobriety for 2 weeks” |
Durkee et al. 2013 (120) | AC (fractional anisotropy) | AUD/AUD+PTSD < controls | 19 AUD (14 male; mean age=32.6, SD=7; 10 smokers, 1 cocaine use disorder, 1 cannabis use disorder, 2 major depressive disorder; 16 mean days abstinent before scan); 17 AUD+PTSD (9 male; mean age=37, SD=8.6; 11 smokers, 2 cocaine use disorder, 1 cannabis use disorder, 3 major depressive disorder; 24 mean days abstinent before scan) | 19 controls (10 male; mean age=26, SD=4; 0 smokers) | Positive urine screen for drugs or positive breath alcohol reading at time of study; other axis I disorders not an exclusion |
Harris et al. 2008 (91) | R lOFC, R cingulum in R dACC (fractional anisotropy) | AUD < controls | 15 AUD (15 male; mean age=48, SD=13; 3 with history of tobacco dependence; abstinent minimum 4 weeks before MRI) | 15 controls (15 male; mean age=56, SD=9); similar to AUD group on depression and anxiety measures | History of depression, schizophrenia, or other SUD |
Hudkins et al. 2012 (86) | B cingulum, genu of CC, L internal capsule (fractional anisotropy) | Nicotine use disorder > controls | 18 nicotine use disorder (10 male; mean age=33.7, SD=7.9) | 18 controls (9 male; mean age=33, SD=10) | Any axis I disorder, dependence on alcohol or drug of abuse, positive drug test on day of scan |
L internal capsule, R cingulum (fractional anisotropy) | Negative correlation with level of dependencec (higher dependence < lower dependence) | ||||
R ACR, R cingulum (fractional anisotropy) | Negative correlation with cigarettes/day (more cigarettes < fewer cigarettes) | ||||
Lane et al. 2010 (83) | B ACR (fractional anisotropy) | Cocaine use disorder < controls | 15 cocaine use disorder (10 male; mean age=38.47, SD=2; 13 smokers, 9 other SUD [sedative 1, opiate 2, cannabis 5, hallucinogens 1, PCP 1, stimulant 2, alcohol 4]) | 18 controls (9 male; mean age=35, SD=2.6; 4 smokers, 0 other SUD) | Comorbid alcohol dependence, positive urine drug test on day of scan, DSM IV axis I disorders other than SUD |
Anterior body of CC (fractional anisotropy, mean λ2 λ3) | Cocaine use disorder < controls | ||||
Lin et al. 2013 (87) | L genu of CC, L rostral body of CC (fractional anisotropy, axial diffusivity, radial diffusivity) | Heavy smokers < controls; positive correlation between years of regular smoking and diffusivity (radial diffusivity and mean diffusivity, not fractional anisotropy) (more smoking < less smoking) | 34 heavy smokers (27 male; mean age=47, SD=7) | 34 nonsmokers (28 male; mean age=47, SD=9) | Drug abuse or dependence, psychiatric disease (Mini International Neuropsychiatric Interview); no subjects were daily drinkers, had had social consequences of drinking, or had difficulty stopping drinking |
Pfefferbaum et al. 2006 (90) | Genu of CC, body of CC (mean diffusivity) | AUD < controls | 57 AUD (age stratified by gender: 40 male [mean age=53, SD=10], 17 female [mean age=50, SD=10]); recruited from rehab centers; mean days abstinent 92; 8 anxiety disorder, 15 mood disorder, 17 depressive disorders, 26 smokers | 74 controls (age stratified by gender: 32 male [mean age=52, SD=14], 42 female [mean age=55, SD=12]; 2 smokers) | DSM IV axis I diagnosis of bipolar disorder or schizophrenia, history of non-alcohol substance dependence |
Qiu et al. 2013 (89) | R OFC, CC, B thalamic radiation (fractional anisotropy) | Opioid use disorder < controls | 18 short-duration opioid use disorder (18 male; mean age=35, SD=8; heroin use duration <10 years); 18 long-duration opioid use disorder (18 male; mean age=38, SD=4; heroin use duration 10–20 years); all regular smokers | 16 controls (16 male; mean age=38, SD=4; all regular smokers) | Schizophrenia, bipolar disorder, ever used any other types of drugs |
B UF (radial diffusivity) | Opioid use disorder < controls | ||||
R OFC (fractional anisotropy) | Negative correlation with length of drug dependence (more years < fewer years) | ||||
Sorg et al. 2012 (82) | Frontal forceps, genu of CC, anterior body of CC, B UF, L anterior internal capsule (fractional anisotropy, axial diffusivity, radial diffusivity) | AUD who relapsed < AUD who remained abstinent | 29 AUD, abstinent at 6-month follow up (minimum 2 weeks sobriety) (28 male; mean age=48, SD=7; inpatients; 21 smokers, 8 past SUD; mean BDI score=8, SD=7); 16 AUD who returned to heavy use (minimum 2 weeks sobriety) (16 male; mean age=48, SD=10; inpatients; 15 smokers, 2 past SUD; mean BDI score=11, SD=8) | 30 controls (29 male; mean age=49, SD=10; 3 smokers; mean BDI score=3, SD=2; no history of axis I disorder) | DSM-IV diagnosis of non-alcohol SUD, hospitalization for a psychiatric condition that preceded AUD |
Upadhyay et al. 2010 (78) | Ventral amygdalofugal pathway, B UF, B internal capsule, B external capsule, genu of CC, anterior midbody of CC, B anterior thalamic radiation (fractional anisotropy) | Opioid use disorder < controls | 10 opioid use disorder (prescription opioid dependence; 7 male; mean age=29, SD=9; 0 smokers) | 10 controls (7 male; mean age=30, SD=8; 0 smokers) | Positive urine screen at time of scan, chronic pain, dependence on other drugs including heroin and alcohol, other psychiatric disorders |
Viswanath et al. 2015 (84) | CC, B internal capsule, B corona radiata (fractional anisotropy) | SUD < psychiatric controls | 99 SUD (alcohol, tobacco, cannabis most prevalent; 64 male; mean age=30, SD=1; inpatients; mean average length of stay=45 days, SD=1; 40% depression, 53% anxiety, 14% bipolar, 37% personality disorder) | 52 psychiatric controls (21 male; mean age=33, SD=2; 38% depression, 50% anxiety, 17% bipolar, 41% personality disorder) | |
Yeh et al. 2009 (85) | R ACR, genu of CC, B UF, B internal capsule, B cingulum, R extreme capsule (fractional anisotropy) | AUD < controls | 10 AUD (10 male; mean age=47, SD=7.6; mean days of abstinence=6, SD=3;10 chronic smokers, 2 with alcohol-induced mood disorder with depressive features, 1 with alcohol-induced psychotic disorder) | 10 controls (10 male; mean age=42.7, SD=9.4; light drinkers, 2 smokers) | General psychiatric conditions, SUD other than nicotine |
Genu of CC (mean diffusivity) | Negative correlation with increased drinking (more drinks < fewer drinks) |
fMRI Tasks of Emotional Regulation
Affect intensity/reactivity.
Affective modulation.
Cognitive modulation.
Behavioral control.
Summary
Resting State Functional and Structural Connectivity
Potential Treatment Targets
Routes of Dysfunction
Impaired functioning in rACC/vmPFC, dACC, lPFC.
Decreased resting state functional and structural connectivity between amygdala/insula and PFC.
Hyperactivation in default mode network during rest/baseline.
Treatment Implications
Augment PFC activation during emotion regulation tasks.
Improve white matter tract integrity or resting state connectivity strength.
Decrease default mode network activation at rest or increase deactivation during tasks.
Limitations and Future Work
Supplementary Material
- View/Download
- 79.30 KB
References
Information & Authors
Information
Published In
History
Authors
Funding Information
Metrics & Citations
Metrics
Citations
Export Citations
If you have the appropriate software installed, you can download article citation data to the citation manager of your choice. Simply select your manager software from the list below and click Download.
For more information or tips please see 'Downloading to a citation manager' in the Help menu.
View Options
View options
PDF/EPUB
View PDF/EPUBLogin options
Already a subscriber? Access your subscription through your login credentials or your institution for full access to this article.
Personal login Institutional Login Open Athens loginNot a subscriber?
PsychiatryOnline subscription options offer access to the DSM-5-TR® library, books, journals, CME, and patient resources. This all-in-one virtual library provides psychiatrists and mental health professionals with key resources for diagnosis, treatment, research, and professional development.
Need more help? PsychiatryOnline Customer Service may be reached by emailing [email protected] or by calling 800-368-5777 (in the U.S.) or 703-907-7322 (outside the U.S.).