Prenatal Expression Patterns of Genes Associated With Neuropsychiatric Disorders
Abstract
Objective
Method
Results
Conclusions
Method
Gene Set Assignment
Statistical Methods

Results
Fetal Effect Analysis
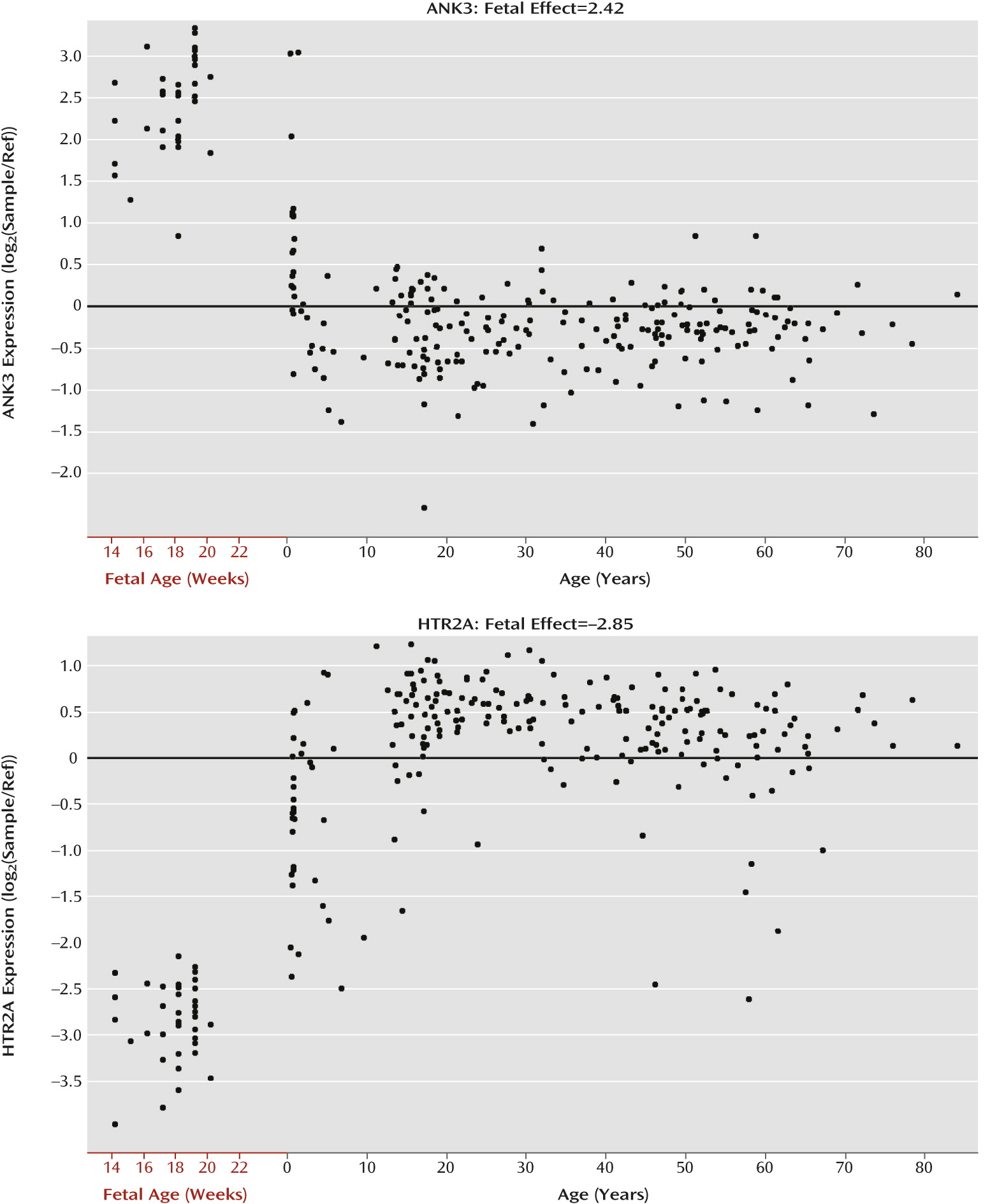
Gene Set | N of Genes | N of Genes Mapped to a Probe | % of Genes in Gene Set with Fetal Effect >0 | p | % of Genes in Gene Set with Fetal Effect >0.5 | p (Two-Sided) | % of Genes in Gene Set with Fetal Effect >1 | p | % of Genes in Gene Set with Fetal Effect >1.5 | p |
---|---|---|---|---|---|---|---|---|---|---|
Genome-wide | 17,678 | 43 | 25 | 12 | 5 | |||||
Neurodevelopmental disorders | 33 | 31 | 65 | 1.13E−02 | 52 | 3.37E−03 | 23 | 1.50E−01 | 16 | 9.64E−02 |
Schizophrenia (SRF) Meta-analysis | 40 | 36 | 42 | 8.95E−01 | 22 | 6.57E−01 | 8 | 4.57E−01 | 3 | 3.87E−01 |
Schizophrenia (PGC) GWAS | 132 | 106 | 50 | 1.13E−01 | 29 | 4.58E−01 | 15 | 3.21E−01 | 6 | 8.02E−01 |
Schizophrenia CNV | 154 | 113 | 41 | 6.58E−01 | 26 | 9.29E−01 | 11 | 6.94E−01 | 4 | 7.09E−01 |
Schizophrenia SNV/INDEL | 240 | 212 | 48 | 1.54E−01 | 29 | 2.06E−01 | 15 | 1.75E−01 | 6 | 7.47E−01 |
ASD CNV | 216 | 176 | 41 | 5.57E−01 | 23 | 5.60E−01 | 9 | 2.30E−01 | 3 | 2.11E−01 |
ASD database | 262 | 242 | 49 | 4.58E−02 | 36 | 3.45E−04 | 19 | 4.06E−03 | 11 | 2.98E−03 |
Bipolar disorder GWAS | 146 | 123 | 38 | 3.00E−01 | 24 | 8.15E−01 | 11 | 8.95E−01 | 6 | 7.95E−01 |
Intellectual disability | 94 | 88 | 56 | 1.46E−02 | 40 | 5.53E−03 | 22 | 2.50E−02 | 13 | 3.70E−02 |
Neurodegenerative disorders | 59 | 46 | 28 | 2.90E−02 | 11 | 1.67E−03 | 4 | 1.37E−02 | 0 | |
Overlap across all categories | 62 | 45 | 7.03E−01 | 31 | 3.61E−01 | 16 | 3.50E−01 | 11 | 1.26E−01 | |
Schizophrenia/ASD CNV overlap | 73 | 45 | 6.74E−01 | 26 | 8.87E−01 | 8 | 2.71E−01 | 4 | 6.55E−01 |
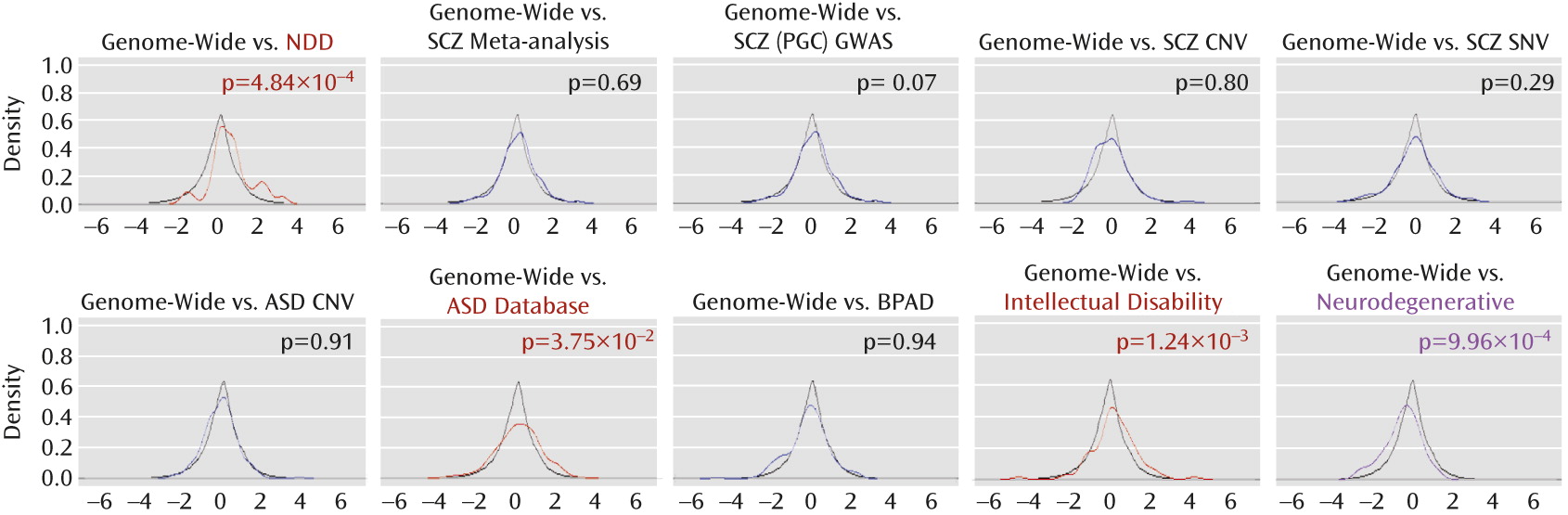
Functional Analysis of Prenatally Enriched Genes
Secondary Analysis of CNV Regions
CNV Loci Associated With ASD and Schizophrenia | ||||
---|---|---|---|---|
Locus | CNV Type | Gene | Fetal Effect | p |
1q21.1 | Dup/Del | NBPF13P | n/a | |
PRKAB2 | 1.39 | 8.97E−57 | ||
FMO5 | ||||
CHD1L | n/a | |||
BCL9 | 2.02 | 2.50E−63 | ||
ACP6 | n/a | |||
GJA5 | 0.25 | 2.82E−03 | ||
GJA8 | −0.49 | 1.40E−09 | ||
GPR89B | n/a | |||
GPR89C | n/a | |||
PDZK1P1 | n/a | |||
NBPF8 | n/a | |||
NBPF24 | n/a | |||
NBPF11 | n/a | |||
NBPF10 | n/a | |||
2p16.3 | Dup/Del | NRXN1 | 1.31 | 6.02E−34 |
15q13.3 | Del/Dup | CHRFAM7A | −1.54 | 5.29E−32 |
ARHGAP11B | n/a | |||
MTMR15 | 0.50 | 1.10E−12 | ||
MTMR10 | n/a | |||
TRPM1 | n/a | |||
MIR211 | n/a | |||
KLF13 | −0.53 | 2.04E−09 | ||
OTUD7A | −0.96 | 6.01E−21 | ||
| | CHRNA7 | 0.83 | 4.76E−08 |
16p11.2 | Del/Dup | SPN | 0.15 | 7.88E−04 |
QPRT | −0.86 | 3.41E−22 | ||
C16orf54 | −0.01 | 9.09E−01 | ||
ZG16 | n/a | |||
KIF22 | 0.22 | 4.67E−04 | ||
MAZ | −0.06 | 7.63E−02 | ||
PRRT2 | −0.57 | 3.02E−13 | ||
PAGR1 (C16orf53) | 1.29 | 1.49E−51 | ||
MVP | −1.16 | 3.84E−28 | ||
CDIPT | −0.59 | 9.04E−32 | ||
SEZ6L2 | −1.44 | 1.83E−42 | ||
ASPHD1 | 0.74 | 1.69E−15 | ||
KCTD13 | 0.16 | 1.68E−02 | ||
TMEM219 | −0.95 | 1.04E−47 | ||
TAOK2 | 0.50 | 7.67E−07 | ||
HIRIP3 | 0.16 | 2.33E−02 | ||
INO80E | −0.03 | 4.98E−01 | ||
DOC2A | −0.64 | 3.67E−30 | ||
C16orf92 | n/a | |||
FAM57B | −0.64 | 3.67E−30 | ||
ALDOA | 0.32 | 1.08E−06 | ||
PPP4C | 1.29 | 1.69E−49 | ||
TBX6 | −0.78 | 1.59E−18 | ||
YPEL3 | −0.95 | 1.35E−39 | ||
GDPD3 | 0.24 | 2.03E−05 | ||
MAPK3 | −1.22 | 2.91E−55 | ||
| | CORO1A | −0.97 | 5.34E−40 |
22q11.21 | Del/Dup | DGCR6 | −0.73 | 2.53E−31 |
PRODH | −1.01 | 6.36E−17 | ||
DGCR5 | 0.65 | 4.41E−13 | ||
DGCR9 | -0.60 | 3.13E−10 | ||
DGCR10 | 0.56 | 3.37E−05 | ||
DGCR2 | −0.73 | 2.09E−28 | ||
DGCR11 | 0.00 | 9.72E−01 | ||
DGCR14 | 0.45 | 1.60E−07 | ||
TSSK2 | −0.55 | 9.14E−08 | ||
GSC2 | n/a | |||
SLC25A1 | 0.48 | 2.19E−15 | ||
CLTCL1 | 0.48 | 2.19E−15 | ||
HIRA | 0.58 | 9.08E−24 | ||
MRPL40 | −0.50 | 1.74E−13 | ||
C22orf39 | n/a | |||
UFD1L | 0.25 | 5.60E−07 | ||
CDC45L | 3.89 | 1.59E−68 | ||
CLDN5 | n/a | |||
SEPT5 | ||||
GP1BB | n/a | |||
TBX1 | −0.70 | 6.57E−25 | ||
GNB1L | -0.25 | 1.14E−04 | ||
C22orf29 | 0.07 | 1.70E−01 | ||
TXNRD2 | −0.84 | 9.34E−41 | ||
COMT | −0.28 | 2.66E−07 | ||
ARVCF | 0.57 | 4.53E−21 | ||
C22orf25 | −1.47 | 8.40E−63 | ||
MIR185 | n/a | |||
DGCR8 | 0.82 | 1.92E−09 | ||
MIR3618 | n/a | |||
MIR1306 | n/a | |||
TRMT2A | −0.40 | 1.14E−08 | ||
RANBP1 | 0.40 | 4.51E−16 | ||
ZDHHC8 | −0.16 | 4.09E−04 | ||
RTN4R | −0.81 | 5.47E−18 | ||
MIR1286 | n/a | |||
DGCR6L | −0.74 | 4.76E−32 | ||
TMEM191B | n/a | |||
RIMBP3 | n/a | |||
ZNF74 | 1.57 | 2.05E−62 | ||
SCARF2 | −0.17 | 2.92E−03 | ||
KLHL22 | 0.38 | 5.11E−16 | ||
MED15 | −0.49 | 1.36E−21 | ||
PI4KA | 0.50 | 2.81E−08 | ||
SERPIND1 | 0.74 | 2.23E−09 | ||
SNAP29 | −0.69 | 6.90E−19 | ||
CRKL | 0.05 | 3.49E−01 | ||
AIFM3 | n/a | |||
LZTR1 | 0.56 | 1.98E−22 | ||
THAP7 | −0.13 | 7.74E−04 | ||
P2RX6 | 0.16 | 2.00E−03 | ||
SLC7A4 | −0.83 | 1.97E−26 | ||
HIC2 | 2.32 | 1.97E−26 |
Locus | CNV Type | Genes | Fetal Effect | p |
---|---|---|---|---|
1q21.1 | Del | HFE2 | 0.09 | 4.82E−01 |
TXNIP | 0.86 | 2.33E−09 | ||
POLR3GL | 0.05 | 2.97E−01 | ||
ANKRD34A | −0.98 | 5.24E−33 | ||
LIX1L | 1.38 | 6.15E−59 | ||
RBM8A | 0.90 | 2.48E−37 | ||
GNRHR2 | −0.49 | 3.85E−07 | ||
PEX11B | −0.70 | 1.44E−36 | ||
ITGA10 | n/a | |||
ANKRD35 | −0.61 | 2.04E−14 | ||
PIAS3 | 0.07 | 1.46E−01 | ||
NUDT17 | −0.30 | 3.23E−08 | ||
POLR3C | 0.19 | 6.81E−05 | ||
RNF115 | 0.08 | 2.06E−01 | ||
CD160 | −0.49 | 1.18E−15 | ||
PDZK1 | 0.23 | 3.52E−02 | ||
GPR89A | n/a |
Locus | CNV Type | Gene | Fetal Effect | p |
---|---|---|---|---|
3p14.1 | Del | SUCLG2 | −0.60 | 1.49E−16 |
FAM19A1 | n/a | |||
FAM19A4 | n/a | |||
C3orf64 | ||||
MIR3136 | n/a | |||
TMF1 | 0.24 | 1.03E−01 | ||
UBA3 | 1.38 | 2.01E−56 | ||
ARL6IP5 | −1.02 | 4.03E−40 | ||
LMOD3 | -0.35 | 6.50E−03 | ||
FRMD4B | 1.78 | 1.97E−34 | ||
| | MITF | 0.17 | 3.79E−02 |
7q11.23 | Dup | FZD9 | −0.41 | 3.34E−05 |
BAZ1B | 0.15 | 9.52E−03 | ||
BCL7B | 0.21 | 1.84E−03 | ||
TBL2 | 0.17 | 9.66E−03 | ||
MLXIPL | n/a | |||
VPS37D | 0.81 | 8.78E−26 | ||
DNAJC30 | 0.20 | 5.41E−04 | ||
WBSCR22 | −0.37 | 2.77E−14 | ||
STX1A | −1.52 | 5.20E−49 | ||
MIR4284 | n/a | |||
ABHD11 | 0.04 | 6.41E−01 | ||
CLDN3 | −0.27 | 3.53E−06 | ||
CLDN4 | 0.02 | 8.86E−01 | ||
WBSCR27 | −0.29 | 2.74E−02 | ||
WBSCR28 | 0.19 | 1.21E−02 | ||
ELN | −0.03 | 7.20E−01 | ||
LIMK1 | −0.50 | 2.58E−10 | ||
MIR590 | n/a | |||
LAT2 | −1.22 | 5.75E−15 | ||
RFC2 | 0.83 | 3.10E−39 | ||
CLIP2 | 0.18 | 7.63E−03 | ||
GTF2IRD1 | 1.68 | 1.64E−68 | ||
| | GTF2I | n/a | |
7q31.1 | Del/Dup | DOCK4 | 0.12 | 1.67E−01 |
8p23.3 | Dup | DLGAP2 | −2.22 | 1.96E−66 |
9p24.3 | Del/Dup | FOXD4 | n/a | |
CBWD1 | 0.52 | 2.04E−13 | ||
C9orf66 | −0.38 | 2.01E−07 | ||
| | DOCK8 | −1.52 | 4.19E−18 |
10q11.23-21.1 | Dup | PRKG1 | n/a | |
MIR605 | n/a | |||
CSTF2T | 0.39 | 1.29E−06 | ||
DKK1 | 0.01 | 9.12E−01 | ||
| | MBL2 | 0.23 | 2.37E−02 |
12q24.31 | Dup | TMEM120B | n/a | |
RHOF | −1.58 | 1.67E−63 | ||
SETD1B | 1.30 | 6.47E−58 | ||
HPD | −0.12 | 9.20E−02 | ||
PSMD9 | 0.18 | 1.09E−02 | ||
WDR66 | −0.58 | 5.32E−10 |
Secondary Analysis of Genes in the ASD Database
Secondary Analysis of Genes Associated With Schizophrenia by Exome Sequencing of Rare Single Nucleotide Variants
Discussion
Prenatal Expression of ASD-Associated Genes
Prenatal Expression of Schizophrenia-Associated Genes
Future Transcriptome Investigations
Footnote
Supplementary Material
- View/Download
- 898.94 KB
References
Information & Authors
Information
Published In
History
Authors
Funding Information
Metrics & Citations
Metrics
Citations
Export Citations
If you have the appropriate software installed, you can download article citation data to the citation manager of your choice. Simply select your manager software from the list below and click Download.
For more information or tips please see 'Downloading to a citation manager' in the Help menu.
View Options
View options
PDF/EPUB
View PDF/EPUBLogin options
Already a subscriber? Access your subscription through your login credentials or your institution for full access to this article.
Personal login Institutional Login Open Athens loginNot a subscriber?
PsychiatryOnline subscription options offer access to the DSM-5-TR® library, books, journals, CME, and patient resources. This all-in-one virtual library provides psychiatrists and mental health professionals with key resources for diagnosis, treatment, research, and professional development.
Need more help? PsychiatryOnline Customer Service may be reached by emailing [email protected] or by calling 800-368-5777 (in the U.S.) or 703-907-7322 (outside the U.S.).